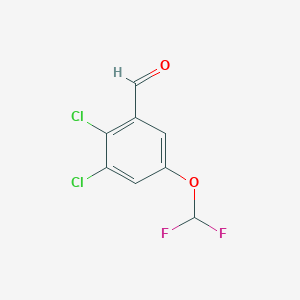
2,3-Dichloro-5-(difluoromethoxy)benzaldehyde
Overview
Description
2,3-Dichloro-5-(difluoromethoxy)benzaldehyde is an organic compound with the molecular formula C8H4Cl2F2O2 It is characterized by the presence of two chlorine atoms, two fluorine atoms, and a methoxy group attached to a benzaldehyde core
Preparation Methods
Synthetic Routes and Reaction Conditions
The synthesis of 2,3-Dichloro-5-(difluoromethoxy)benzaldehyde typically involves the chlorination and fluorination of appropriate benzaldehyde derivatives. One common method involves the reaction of 2,3-dichlorobenzaldehyde with difluoromethyl ether under specific conditions to introduce the difluoromethoxy group. The reaction is usually carried out in the presence of a catalyst and under controlled temperature and pressure to ensure high yield and purity .
Industrial Production Methods
Industrial production of this compound follows similar synthetic routes but on a larger scale. The process involves the use of industrial-grade reagents and catalysts, with stringent control over reaction parameters to optimize yield and minimize by-products. The final product is purified through distillation or recrystallization to achieve the desired purity levels required for commercial applications .
Chemical Reactions Analysis
Types of Reactions
2,3-Dichloro-5-(difluoromethoxy)benzaldehyde undergoes various chemical reactions, including:
Oxidation: The aldehyde group can be oxidized to form the corresponding carboxylic acid.
Reduction: The aldehyde group can be reduced to form the corresponding alcohol.
Substitution: The chlorine atoms can be substituted with other functional groups through nucleophilic substitution reactions.
Common Reagents and Conditions
Oxidation: Common oxidizing agents include potassium permanganate (KMnO4) and chromium trioxide (CrO3).
Reduction: Reducing agents such as sodium borohydride (NaBH4) or lithium aluminum hydride (LiAlH4) are typically used.
Substitution: Nucleophiles such as amines or thiols can be used to replace the chlorine atoms under appropriate conditions.
Major Products Formed
Oxidation: 2,3-Dichloro-5-(difluoromethoxy)benzoic acid.
Reduction: 2,3-Dichloro-5-(difluoromethoxy)benzyl alcohol.
Substitution: Various substituted derivatives depending on the nucleophile used.
Scientific Research Applications
2,3-Dichloro-5-(difluoromethoxy)benzaldehyde has several scientific research applications:
Chemistry: Used as an intermediate in the synthesis of more complex organic molecules.
Biology: Investigated for its potential biological activity and interactions with biomolecules.
Medicine: Explored for its potential use in the development of pharmaceutical compounds.
Industry: Utilized in the production of agrochemicals and other industrial chemicals.
Mechanism of Action
The mechanism of action of 2,3-Dichloro-5-(difluoromethoxy)benzaldehyde involves its interaction with specific molecular targets. The presence of electron-withdrawing groups (chlorine and fluorine) on the benzaldehyde core can influence its reactivity and binding affinity to various enzymes and receptors. These interactions can modulate biological pathways, leading to potential therapeutic effects.
Comparison with Similar Compounds
Similar Compounds
- 2,3-Dichloro-5-(trifluoromethoxy)benzaldehyde
- 2,3-Dichloro-5-(methoxy)benzaldehyde
- 2,3-Dichloro-5-(fluoromethoxy)benzaldehyde
Uniqueness
2,3-Dichloro-5-(difluoromethoxy)benzaldehyde is unique due to the presence of both chlorine and difluoromethoxy groups, which impart distinct chemical and physical properties. The difluoromethoxy group enhances the compound’s lipophilicity and metabolic stability, making it a valuable intermediate in the synthesis of pharmaceuticals and agrochemicals .
Properties
IUPAC Name |
2,3-dichloro-5-(difluoromethoxy)benzaldehyde | |
---|---|---|
Source | PubChem | |
URL | https://pubchem.ncbi.nlm.nih.gov | |
Description | Data deposited in or computed by PubChem | |
InChI |
InChI=1S/C8H4Cl2F2O2/c9-6-2-5(14-8(11)12)1-4(3-13)7(6)10/h1-3,8H | |
Source | PubChem | |
URL | https://pubchem.ncbi.nlm.nih.gov | |
Description | Data deposited in or computed by PubChem | |
InChI Key |
WCAMREOGQVOGEM-UHFFFAOYSA-N | |
Source | PubChem | |
URL | https://pubchem.ncbi.nlm.nih.gov | |
Description | Data deposited in or computed by PubChem | |
Canonical SMILES |
C1=C(C=C(C(=C1C=O)Cl)Cl)OC(F)F | |
Source | PubChem | |
URL | https://pubchem.ncbi.nlm.nih.gov | |
Description | Data deposited in or computed by PubChem | |
Molecular Formula |
C8H4Cl2F2O2 | |
Source | PubChem | |
URL | https://pubchem.ncbi.nlm.nih.gov | |
Description | Data deposited in or computed by PubChem | |
Molecular Weight |
241.02 g/mol | |
Source | PubChem | |
URL | https://pubchem.ncbi.nlm.nih.gov | |
Description | Data deposited in or computed by PubChem | |
Retrosynthesis Analysis
AI-Powered Synthesis Planning: Our tool employs the Template_relevance Pistachio, Template_relevance Bkms_metabolic, Template_relevance Pistachio_ringbreaker, Template_relevance Reaxys, Template_relevance Reaxys_biocatalysis model, leveraging a vast database of chemical reactions to predict feasible synthetic routes.
One-Step Synthesis Focus: Specifically designed for one-step synthesis, it provides concise and direct routes for your target compounds, streamlining the synthesis process.
Accurate Predictions: Utilizing the extensive PISTACHIO, BKMS_METABOLIC, PISTACHIO_RINGBREAKER, REAXYS, REAXYS_BIOCATALYSIS database, our tool offers high-accuracy predictions, reflecting the latest in chemical research and data.
Strategy Settings
Precursor scoring | Relevance Heuristic |
---|---|
Min. plausibility | 0.01 |
Model | Template_relevance |
Template Set | Pistachio/Bkms_metabolic/Pistachio_ringbreaker/Reaxys/Reaxys_biocatalysis |
Top-N result to add to graph | 6 |
Feasible Synthetic Routes
Disclaimer and Information on In-Vitro Research Products
Please be aware that all articles and product information presented on BenchChem are intended solely for informational purposes. The products available for purchase on BenchChem are specifically designed for in-vitro studies, which are conducted outside of living organisms. In-vitro studies, derived from the Latin term "in glass," involve experiments performed in controlled laboratory settings using cells or tissues. It is important to note that these products are not categorized as medicines or drugs, and they have not received approval from the FDA for the prevention, treatment, or cure of any medical condition, ailment, or disease. We must emphasize that any form of bodily introduction of these products into humans or animals is strictly prohibited by law. It is essential to adhere to these guidelines to ensure compliance with legal and ethical standards in research and experimentation.