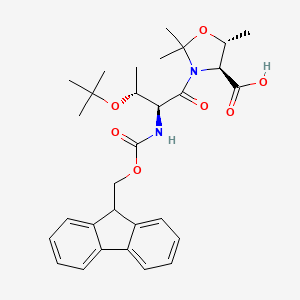
Fmoc-Thr(tBu)-Thr(Psi(Me,Me)pro)-OH
Overview
Description
Fmoc-Thr(tBu)-Thr(Psi(Me,Me)pro)-OH is a synthetic peptide derivative that incorporates fluorenylmethyloxycarbonyl (Fmoc) as a protecting group. This compound is used in peptide synthesis, particularly in the solid-phase synthesis of peptides. The presence of tert-butyl (tBu) and Psi(Me,Me)pro groups provides steric hindrance and stability, making it a valuable building block in the synthesis of complex peptides.
Preparation Methods
Synthetic Routes and Reaction Conditions
The synthesis of Fmoc-Thr(tBu)-Thr(Psi(Me,Me)pro)-OH typically involves solid-phase peptide synthesis (SPPS) using Fmoc/tBu chemistry. The process begins with the attachment of the first amino acid to a solid resin, followed by sequential addition of protected amino acids. Each amino acid addition involves deprotection of the Fmoc group using piperidine, coupling of the next amino acid using activating agents like HBTU or DIC, and washing steps to remove excess reagents .
Industrial Production Methods
In industrial settings, the synthesis of this compound follows similar SPPS protocols but on a larger scale. Automated peptide synthesizers are often used to streamline the process, ensuring high yields and purity. The use of greener solvents and optimized reaction conditions is becoming more prevalent to reduce environmental impact .
Chemical Reactions Analysis
Types of Reactions
Fmoc-Thr(tBu)-Thr(Psi(Me,Me)pro)-OH undergoes various chemical reactions, including:
Deprotection: Removal of the Fmoc group using piperidine.
Coupling: Formation of peptide bonds using activating agents like HBTU or DIC.
Cleavage: Removal of the peptide from the solid support using TFA (trifluoroacetic acid).
Common Reagents and Conditions
Deprotection: Piperidine in DMF (dimethylformamide).
Coupling: HBTU or DIC in the presence of a base like DIPEA (N,N-diisopropylethylamine).
Cleavage: TFA with scavengers like water, TIS (triisopropylsilane), and EDT (ethanedithiol).
Major Products Formed
The primary product formed is the desired peptide sequence, with side products including truncated peptides and deletion sequences, which are minimized through optimized reaction conditions .
Scientific Research Applications
Fmoc-Thr(tBu)-Thr(Psi(Me,Me)pro)-OH is widely used in scientific research for the synthesis of peptides with specific sequences and functionalities. Its applications include:
Chemistry: Synthesis of complex peptides and peptidomimetics for studying structure-activity relationships.
Biology: Creation of peptide-based probes and inhibitors for studying biological pathways.
Medicine: Development of peptide therapeutics and vaccines.
Industry: Production of peptides for use in cosmetics, food additives, and materials science
Mechanism of Action
The mechanism of action of Fmoc-Thr(tBu)-Thr(Psi(Me,Me)pro)-OH involves its role as a building block in peptide synthesis. The Fmoc group protects the amino terminus during synthesis, preventing unwanted side reactions. The tert-butyl and Psi(Me,Me)pro groups provide steric hindrance, enhancing the stability and solubility of the peptide. These features facilitate the efficient assembly of complex peptide sequences .
Comparison with Similar Compounds
Similar Compounds
Fmoc-Ser(tBu)-OH: Similar protecting groups but with serine instead of threonine.
Fmoc-Val-OH: Lacks the tert-butyl and Psi(Me,Me)pro groups, making it less sterically hindered.
Fmoc-Gly-OH: Simplest Fmoc-protected amino acid without additional protecting groups.
Uniqueness
Fmoc-Thr(tBu)-Thr(Psi(Me,Me)pro)-OH is unique due to its combination of protecting groups that provide both steric hindrance and stability. This makes it particularly useful in the synthesis of peptides that require precise control over their conformation and stability .
Properties
IUPAC Name |
(4S,5R)-3-[(2S,3R)-2-(9H-fluoren-9-ylmethoxycarbonylamino)-3-[(2-methylpropan-2-yl)oxy]butanoyl]-2,2,5-trimethyl-1,3-oxazolidine-4-carboxylic acid | |
---|---|---|
Source | PubChem | |
URL | https://pubchem.ncbi.nlm.nih.gov | |
Description | Data deposited in or computed by PubChem | |
InChI |
InChI=1S/C30H38N2O7/c1-17(38-29(3,4)5)24(26(33)32-25(27(34)35)18(2)39-30(32,6)7)31-28(36)37-16-23-21-14-10-8-12-19(21)20-13-9-11-15-22(20)23/h8-15,17-18,23-25H,16H2,1-7H3,(H,31,36)(H,34,35)/t17-,18-,24+,25+/m1/s1 | |
Source | PubChem | |
URL | https://pubchem.ncbi.nlm.nih.gov | |
Description | Data deposited in or computed by PubChem | |
InChI Key |
CYPAPDDRSYFIHL-GEUPUMGHSA-N | |
Source | PubChem | |
URL | https://pubchem.ncbi.nlm.nih.gov | |
Description | Data deposited in or computed by PubChem | |
Canonical SMILES |
CC1C(N(C(O1)(C)C)C(=O)C(C(C)OC(C)(C)C)NC(=O)OCC2C3=CC=CC=C3C4=CC=CC=C24)C(=O)O | |
Source | PubChem | |
URL | https://pubchem.ncbi.nlm.nih.gov | |
Description | Data deposited in or computed by PubChem | |
Isomeric SMILES |
C[C@@H]1[C@H](N(C(O1)(C)C)C(=O)[C@H]([C@@H](C)OC(C)(C)C)NC(=O)OCC2C3=CC=CC=C3C4=CC=CC=C24)C(=O)O | |
Source | PubChem | |
URL | https://pubchem.ncbi.nlm.nih.gov | |
Description | Data deposited in or computed by PubChem | |
Molecular Formula |
C30H38N2O7 | |
Source | PubChem | |
URL | https://pubchem.ncbi.nlm.nih.gov | |
Description | Data deposited in or computed by PubChem | |
Molecular Weight |
538.6 g/mol | |
Source | PubChem | |
URL | https://pubchem.ncbi.nlm.nih.gov | |
Description | Data deposited in or computed by PubChem | |
Retrosynthesis Analysis
AI-Powered Synthesis Planning: Our tool employs the Template_relevance Pistachio, Template_relevance Bkms_metabolic, Template_relevance Pistachio_ringbreaker, Template_relevance Reaxys, Template_relevance Reaxys_biocatalysis model, leveraging a vast database of chemical reactions to predict feasible synthetic routes.
One-Step Synthesis Focus: Specifically designed for one-step synthesis, it provides concise and direct routes for your target compounds, streamlining the synthesis process.
Accurate Predictions: Utilizing the extensive PISTACHIO, BKMS_METABOLIC, PISTACHIO_RINGBREAKER, REAXYS, REAXYS_BIOCATALYSIS database, our tool offers high-accuracy predictions, reflecting the latest in chemical research and data.
Strategy Settings
Precursor scoring | Relevance Heuristic |
---|---|
Min. plausibility | 0.01 |
Model | Template_relevance |
Template Set | Pistachio/Bkms_metabolic/Pistachio_ringbreaker/Reaxys/Reaxys_biocatalysis |
Top-N result to add to graph | 6 |
Feasible Synthetic Routes
Disclaimer and Information on In-Vitro Research Products
Please be aware that all articles and product information presented on BenchChem are intended solely for informational purposes. The products available for purchase on BenchChem are specifically designed for in-vitro studies, which are conducted outside of living organisms. In-vitro studies, derived from the Latin term "in glass," involve experiments performed in controlled laboratory settings using cells or tissues. It is important to note that these products are not categorized as medicines or drugs, and they have not received approval from the FDA for the prevention, treatment, or cure of any medical condition, ailment, or disease. We must emphasize that any form of bodily introduction of these products into humans or animals is strictly prohibited by law. It is essential to adhere to these guidelines to ensure compliance with legal and ethical standards in research and experimentation.