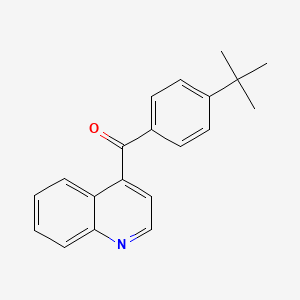
4-(4-tert-Butylbenzoyl)quinoline
Overview
Description
4-(4-tert-Butylbenzoyl)quinoline is a synthetic compound that belongs to the class of quinoline derivatives. It is known for its unique chemical structure and biological activity, making it widely used in various fields, including medical, environmental, and industrial research.
Preparation Methods
Synthetic Routes and Reaction Conditions
The synthesis of 4-(4-tert-Butylbenzoyl)quinoline can be achieved through various methods. One common approach involves the reaction between 4-tert-butylbenzoyl chloride and quinoline under specific conditions . Another method includes the use of activated alkyne-based reactions, K2CO3/DIPEA-catalyzed reactions, and Pd-catalyzed Buchwald–Hartwig amination .
Industrial Production Methods
Industrial production of this compound typically involves large-scale synthesis using optimized reaction conditions to ensure high yield and purity. The use of recyclable catalysts and green chemistry principles is often employed to make the process more sustainable .
Chemical Reactions Analysis
Types of Reactions
4-(4-tert-Butylbenzoyl)quinoline undergoes various chemical reactions, including:
Oxidation: This reaction involves the addition of oxygen or the removal of hydrogen.
Reduction: This reaction involves the addition of hydrogen or the removal of oxygen.
Substitution: This reaction involves the replacement of one atom or group of atoms with another.
Common Reagents and Conditions
Common reagents used in these reactions include oxidizing agents like potassium permanganate, reducing agents like sodium borohydride, and various nucleophiles for substitution reactions .
Major Products Formed
The major products formed from these reactions depend on the specific conditions and reagents used. For example, oxidation may yield quinoline N-oxide derivatives, while reduction may produce quinoline derivatives with reduced functional groups .
Scientific Research Applications
4-(4-tert-Butylbenzoyl)quinoline has a wide range of scientific research applications:
Chemistry: It is used as a building block for the synthesis of more complex molecules.
Biology: It is studied for its potential biological activities, including antibacterial and anticancer properties.
Medicine: It is explored for its potential therapeutic applications, particularly in the development of new drugs.
Industry: It is used in the production of various industrial chemicals and materials.
Mechanism of Action
The mechanism of action of 4-(4-tert-Butylbenzoyl)quinoline involves its interaction with specific molecular targets and pathways. For instance, quinoline derivatives are known to target bacterial type II topoisomerases, such as gyrase and topoisomerase IV, converting them into toxic enzymes that fragment the bacterial chromosome . This mechanism is crucial for its antibacterial activity.
Comparison with Similar Compounds
Similar Compounds
Some similar compounds include:
Uniqueness
4-(4-tert-Butylbenzoyl)quinoline is unique due to its specific tert-butylbenzoyl group, which imparts distinct chemical and biological properties. This uniqueness makes it a valuable compound for various research and industrial applications.
Properties
IUPAC Name |
(4-tert-butylphenyl)-quinolin-4-ylmethanone | |
---|---|---|
Source | PubChem | |
URL | https://pubchem.ncbi.nlm.nih.gov | |
Description | Data deposited in or computed by PubChem | |
InChI |
InChI=1S/C20H19NO/c1-20(2,3)15-10-8-14(9-11-15)19(22)17-12-13-21-18-7-5-4-6-16(17)18/h4-13H,1-3H3 | |
Source | PubChem | |
URL | https://pubchem.ncbi.nlm.nih.gov | |
Description | Data deposited in or computed by PubChem | |
InChI Key |
LDVVLCORKHNFHI-UHFFFAOYSA-N | |
Source | PubChem | |
URL | https://pubchem.ncbi.nlm.nih.gov | |
Description | Data deposited in or computed by PubChem | |
Canonical SMILES |
CC(C)(C)C1=CC=C(C=C1)C(=O)C2=CC=NC3=CC=CC=C23 | |
Source | PubChem | |
URL | https://pubchem.ncbi.nlm.nih.gov | |
Description | Data deposited in or computed by PubChem | |
Molecular Formula |
C20H19NO | |
Source | PubChem | |
URL | https://pubchem.ncbi.nlm.nih.gov | |
Description | Data deposited in or computed by PubChem | |
Molecular Weight |
289.4 g/mol | |
Source | PubChem | |
URL | https://pubchem.ncbi.nlm.nih.gov | |
Description | Data deposited in or computed by PubChem | |
Retrosynthesis Analysis
AI-Powered Synthesis Planning: Our tool employs the Template_relevance Pistachio, Template_relevance Bkms_metabolic, Template_relevance Pistachio_ringbreaker, Template_relevance Reaxys, Template_relevance Reaxys_biocatalysis model, leveraging a vast database of chemical reactions to predict feasible synthetic routes.
One-Step Synthesis Focus: Specifically designed for one-step synthesis, it provides concise and direct routes for your target compounds, streamlining the synthesis process.
Accurate Predictions: Utilizing the extensive PISTACHIO, BKMS_METABOLIC, PISTACHIO_RINGBREAKER, REAXYS, REAXYS_BIOCATALYSIS database, our tool offers high-accuracy predictions, reflecting the latest in chemical research and data.
Strategy Settings
Precursor scoring | Relevance Heuristic |
---|---|
Min. plausibility | 0.01 |
Model | Template_relevance |
Template Set | Pistachio/Bkms_metabolic/Pistachio_ringbreaker/Reaxys/Reaxys_biocatalysis |
Top-N result to add to graph | 6 |
Feasible Synthetic Routes
Disclaimer and Information on In-Vitro Research Products
Please be aware that all articles and product information presented on BenchChem are intended solely for informational purposes. The products available for purchase on BenchChem are specifically designed for in-vitro studies, which are conducted outside of living organisms. In-vitro studies, derived from the Latin term "in glass," involve experiments performed in controlled laboratory settings using cells or tissues. It is important to note that these products are not categorized as medicines or drugs, and they have not received approval from the FDA for the prevention, treatment, or cure of any medical condition, ailment, or disease. We must emphasize that any form of bodily introduction of these products into humans or animals is strictly prohibited by law. It is essential to adhere to these guidelines to ensure compliance with legal and ethical standards in research and experimentation.