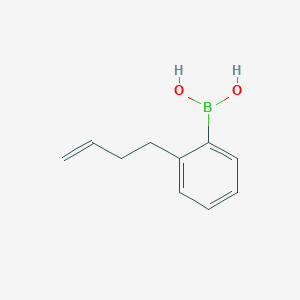
(2-(But-3-en-1-yl)phenyl)boronic acid
Overview
Description
(2-(But-3-en-1-yl)phenyl)boronic acid is an organoboron compound that features a phenyl ring substituted with a but-3-en-1-yl group and a boronic acid functional group. This compound is of significant interest in organic chemistry due to its versatility in various chemical reactions, particularly in the formation of carbon-carbon bonds through Suzuki-Miyaura coupling reactions.
Preparation Methods
Synthetic Routes and Reaction Conditions: The synthesis of (2-(But-3-en-1-yl)phenyl)boronic acid typically involves the hydroboration of an appropriate alkyne or alkene precursor. One common method is the hydroboration of 1-bromo-2-(but-3-en-1-yl)benzene with a borane reagent, followed by oxidation to yield the boronic acid. The reaction conditions often include the use of a solvent such as tetrahydrofuran (THF) and a catalyst like palladium.
Industrial Production Methods: Industrial production of this compound may involve similar synthetic routes but on a larger scale. The process is optimized for yield and purity, often employing continuous flow reactors and automated systems to ensure consistent production. The use of environmentally benign solvents and reagents is also a consideration in industrial settings.
Chemical Reactions Analysis
Types of Reactions: (2-(But-3-en-1-yl)phenyl)boronic acid undergoes various types of chemical reactions, including:
Oxidation: The boronic acid group can be oxidized to form boronic esters or borates.
Reduction: Reduction reactions can convert the boronic acid to the corresponding borane.
Substitution: The compound can participate in nucleophilic substitution reactions, where the boronic acid group is replaced by other functional groups.
Common Reagents and Conditions:
Oxidation: Hydrogen peroxide or other oxidizing agents in the presence of a base.
Reduction: Sodium borohydride or lithium aluminum hydride.
Substitution: Halogenated reagents or organometallic compounds under palladium-catalyzed conditions.
Major Products:
Oxidation: Boronic esters or borates.
Reduction: Boranes.
Substitution: Various substituted phenyl derivatives.
Scientific Research Applications
(2-(But-3-en-1-yl)phenyl)boronic acid has numerous applications in scientific research:
Chemistry: It is widely used in Suzuki-Miyaura coupling reactions to form carbon-carbon bonds, which are essential in the synthesis of complex organic molecules.
Biology: The compound can be used to modify biomolecules, such as proteins and nucleic acids, through boronate ester formation.
Industry: The compound is used in the production of advanced materials, including polymers and electronic components.
Mechanism of Action
The mechanism by which (2-(But-3-en-1-yl)phenyl)boronic acid exerts its effects involves the formation of boronate complexes. The boronic acid group can interact with diols and other Lewis bases to form stable cyclic boronate esters. This interaction is crucial in various catalytic processes, including the Suzuki-Miyaura coupling, where the boronic acid transfers its organic group to a palladium catalyst, facilitating the formation of a new carbon-carbon bond.
Comparison with Similar Compounds
Phenylboronic acid: Lacks the but-3-en-1-yl group, making it less versatile in certain reactions.
(2-Methylphenyl)boronic acid: Contains a methyl group instead of the but-3-en-1-yl group, affecting its reactivity and applications.
(2-(But-3-en-1-yl)phenyl)boronic ester: The ester form of the compound, which has different solubility and reactivity properties.
Uniqueness: (2-(But-3-en-1-yl)phenyl)boronic acid is unique due to the presence of the but-3-en-1-yl group, which enhances its reactivity and allows for more diverse applications in organic synthesis and material science. This structural feature distinguishes it from other boronic acids and esters, providing unique advantages in specific chemical reactions and industrial processes.
Properties
IUPAC Name |
(2-but-3-enylphenyl)boronic acid | |
---|---|---|
Source | PubChem | |
URL | https://pubchem.ncbi.nlm.nih.gov | |
Description | Data deposited in or computed by PubChem | |
InChI |
InChI=1S/C10H13BO2/c1-2-3-6-9-7-4-5-8-10(9)11(12)13/h2,4-5,7-8,12-13H,1,3,6H2 | |
Source | PubChem | |
URL | https://pubchem.ncbi.nlm.nih.gov | |
Description | Data deposited in or computed by PubChem | |
InChI Key |
DVVHNJPNOJSHDI-UHFFFAOYSA-N | |
Source | PubChem | |
URL | https://pubchem.ncbi.nlm.nih.gov | |
Description | Data deposited in or computed by PubChem | |
Canonical SMILES |
B(C1=CC=CC=C1CCC=C)(O)O | |
Source | PubChem | |
URL | https://pubchem.ncbi.nlm.nih.gov | |
Description | Data deposited in or computed by PubChem | |
Molecular Formula |
C10H13BO2 | |
Source | PubChem | |
URL | https://pubchem.ncbi.nlm.nih.gov | |
Description | Data deposited in or computed by PubChem | |
Molecular Weight |
176.02 g/mol | |
Source | PubChem | |
URL | https://pubchem.ncbi.nlm.nih.gov | |
Description | Data deposited in or computed by PubChem | |
Synthesis routes and methods
Procedure details
Retrosynthesis Analysis
AI-Powered Synthesis Planning: Our tool employs the Template_relevance Pistachio, Template_relevance Bkms_metabolic, Template_relevance Pistachio_ringbreaker, Template_relevance Reaxys, Template_relevance Reaxys_biocatalysis model, leveraging a vast database of chemical reactions to predict feasible synthetic routes.
One-Step Synthesis Focus: Specifically designed for one-step synthesis, it provides concise and direct routes for your target compounds, streamlining the synthesis process.
Accurate Predictions: Utilizing the extensive PISTACHIO, BKMS_METABOLIC, PISTACHIO_RINGBREAKER, REAXYS, REAXYS_BIOCATALYSIS database, our tool offers high-accuracy predictions, reflecting the latest in chemical research and data.
Strategy Settings
Precursor scoring | Relevance Heuristic |
---|---|
Min. plausibility | 0.01 |
Model | Template_relevance |
Template Set | Pistachio/Bkms_metabolic/Pistachio_ringbreaker/Reaxys/Reaxys_biocatalysis |
Top-N result to add to graph | 6 |
Feasible Synthetic Routes
Disclaimer and Information on In-Vitro Research Products
Please be aware that all articles and product information presented on BenchChem are intended solely for informational purposes. The products available for purchase on BenchChem are specifically designed for in-vitro studies, which are conducted outside of living organisms. In-vitro studies, derived from the Latin term "in glass," involve experiments performed in controlled laboratory settings using cells or tissues. It is important to note that these products are not categorized as medicines or drugs, and they have not received approval from the FDA for the prevention, treatment, or cure of any medical condition, ailment, or disease. We must emphasize that any form of bodily introduction of these products into humans or animals is strictly prohibited by law. It is essential to adhere to these guidelines to ensure compliance with legal and ethical standards in research and experimentation.