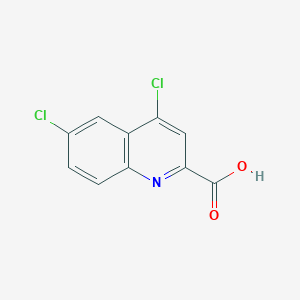
4,6-Dichloro-2-quinolinecarboxylic acid
Overview
Description
4,6-Dichloro-2-quinolinecarboxylic acid is an organic compound with the molecular formula C10H5Cl2NO2. It is a derivative of quinoline, a heterocyclic aromatic organic compound. This compound is known for its applications in various fields, including medicinal chemistry and industrial processes.
Preparation Methods
Synthetic Routes and Reaction Conditions
The synthesis of 4,6-Dichloro-2-quinolinecarboxylic acid typically involves the chlorination of quinoline derivatives. One common method includes the reaction of 2-quinolinecarboxylic acid with chlorine gas under controlled conditions to introduce chlorine atoms at the 4 and 6 positions of the quinoline ring . The reaction is usually carried out in the presence of a catalyst, such as iron(III) chloride, to facilitate the chlorination process.
Industrial Production Methods
Industrial production of this compound follows similar synthetic routes but on a larger scale. The process involves the use of large reactors and precise control of reaction conditions to ensure high yield and purity of the final product. The use of continuous flow reactors and automated systems helps in maintaining consistency and efficiency in production .
Chemical Reactions Analysis
Types of Reactions
4,6-Dichloro-2-quinolinecarboxylic acid undergoes various chemical reactions, including:
Substitution Reactions: The chlorine atoms at the 4 and 6 positions can be substituted with other functional groups through nucleophilic substitution reactions.
Oxidation Reactions: The compound can be oxidized to form quinoline derivatives with different oxidation states.
Reduction Reactions: Reduction of the carboxylic acid group can lead to the formation of corresponding alcohols or aldehydes.
Common Reagents and Conditions
Substitution Reactions: Common reagents include nucleophiles such as amines, thiols, and alkoxides. The reactions are typically carried out in polar aprotic solvents like dimethyl sulfoxide (DMSO) or dimethylformamide (DMF) at elevated temperatures.
Oxidation Reactions: Oxidizing agents such as potassium permanganate (KMnO4) or chromium trioxide (CrO3) are used under acidic or basic conditions.
Reduction Reactions: Reducing agents like lithium aluminum hydride (LiAlH4) or sodium borohydride (NaBH4) are employed under anhydrous conditions.
Major Products Formed
Substitution Reactions: Products include various substituted quinoline derivatives with different functional groups replacing the chlorine atoms.
Oxidation Reactions: Products include quinoline N-oxides and other oxidized derivatives.
Reduction Reactions: Products include quinoline alcohols and aldehydes.
Scientific Research Applications
4,6-Dichloro-2-quinolinecarboxylic acid has several applications in scientific research:
Medicinal Chemistry: It is used as an intermediate in the synthesis of pharmaceutical compounds, particularly those with antimicrobial and anticancer properties.
Biological Research: The compound is used in studies related to enzyme inhibition and receptor binding due to its ability to interact with biological macromolecules.
Industrial Chemistry: It serves as a precursor for the synthesis of dyes, pigments, and other industrial chemicals.
Mechanism of Action
The mechanism of action of 4,6-Dichloro-2-quinolinecarboxylic acid involves its interaction with specific molecular targets. The compound can act as an inhibitor of certain enzymes by binding to their active sites, thereby preventing substrate binding and subsequent catalytic activity. Additionally, it can interact with cellular receptors, modulating signal transduction pathways and affecting cellular functions .
Comparison with Similar Compounds
Similar Compounds
4,7-Dichloroquinoline: Another chlorinated quinoline derivative used in the synthesis of antimalarial drugs.
Quinclorac: A quinoline derivative used as an herbicide with a similar structure but different functional groups.
Uniqueness
4,6-Dichloro-2-quinolinecarboxylic acid is unique due to its specific substitution pattern, which imparts distinct chemical and biological properties. Its ability to undergo various chemical reactions and its applications in diverse fields make it a valuable compound in both research and industry .
Properties
IUPAC Name |
4,6-dichloroquinoline-2-carboxylic acid | |
---|---|---|
Source | PubChem | |
URL | https://pubchem.ncbi.nlm.nih.gov | |
Description | Data deposited in or computed by PubChem | |
InChI |
InChI=1S/C10H5Cl2NO2/c11-5-1-2-8-6(3-5)7(12)4-9(13-8)10(14)15/h1-4H,(H,14,15) | |
Source | PubChem | |
URL | https://pubchem.ncbi.nlm.nih.gov | |
Description | Data deposited in or computed by PubChem | |
InChI Key |
KBYSXQKAWAUVJZ-UHFFFAOYSA-N | |
Source | PubChem | |
URL | https://pubchem.ncbi.nlm.nih.gov | |
Description | Data deposited in or computed by PubChem | |
Canonical SMILES |
C1=CC2=C(C=C1Cl)C(=CC(=N2)C(=O)O)Cl | |
Source | PubChem | |
URL | https://pubchem.ncbi.nlm.nih.gov | |
Description | Data deposited in or computed by PubChem | |
Molecular Formula |
C10H5Cl2NO2 | |
Source | PubChem | |
URL | https://pubchem.ncbi.nlm.nih.gov | |
Description | Data deposited in or computed by PubChem | |
DSSTOX Substance ID |
DTXSID30733692 | |
Record name | 4,6-Dichloroquinoline-2-carboxylic acid | |
Source | EPA DSSTox | |
URL | https://comptox.epa.gov/dashboard/DTXSID30733692 | |
Description | DSSTox provides a high quality public chemistry resource for supporting improved predictive toxicology. | |
Molecular Weight |
242.05 g/mol | |
Source | PubChem | |
URL | https://pubchem.ncbi.nlm.nih.gov | |
Description | Data deposited in or computed by PubChem | |
CAS No. |
902742-67-0 | |
Record name | 4,6-Dichloroquinoline-2-carboxylic acid | |
Source | EPA DSSTox | |
URL | https://comptox.epa.gov/dashboard/DTXSID30733692 | |
Description | DSSTox provides a high quality public chemistry resource for supporting improved predictive toxicology. | |
Retrosynthesis Analysis
AI-Powered Synthesis Planning: Our tool employs the Template_relevance Pistachio, Template_relevance Bkms_metabolic, Template_relevance Pistachio_ringbreaker, Template_relevance Reaxys, Template_relevance Reaxys_biocatalysis model, leveraging a vast database of chemical reactions to predict feasible synthetic routes.
One-Step Synthesis Focus: Specifically designed for one-step synthesis, it provides concise and direct routes for your target compounds, streamlining the synthesis process.
Accurate Predictions: Utilizing the extensive PISTACHIO, BKMS_METABOLIC, PISTACHIO_RINGBREAKER, REAXYS, REAXYS_BIOCATALYSIS database, our tool offers high-accuracy predictions, reflecting the latest in chemical research and data.
Strategy Settings
Precursor scoring | Relevance Heuristic |
---|---|
Min. plausibility | 0.01 |
Model | Template_relevance |
Template Set | Pistachio/Bkms_metabolic/Pistachio_ringbreaker/Reaxys/Reaxys_biocatalysis |
Top-N result to add to graph | 6 |
Feasible Synthetic Routes
Disclaimer and Information on In-Vitro Research Products
Please be aware that all articles and product information presented on BenchChem are intended solely for informational purposes. The products available for purchase on BenchChem are specifically designed for in-vitro studies, which are conducted outside of living organisms. In-vitro studies, derived from the Latin term "in glass," involve experiments performed in controlled laboratory settings using cells or tissues. It is important to note that these products are not categorized as medicines or drugs, and they have not received approval from the FDA for the prevention, treatment, or cure of any medical condition, ailment, or disease. We must emphasize that any form of bodily introduction of these products into humans or animals is strictly prohibited by law. It is essential to adhere to these guidelines to ensure compliance with legal and ethical standards in research and experimentation.