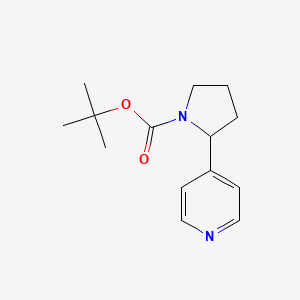
tert-Butyl 2-(pyridin-4-yl)pyrrolidine-1-carboxylate
Overview
Description
Tert-Butyl 2-(pyridin-4-yl)pyrrolidine-1-carboxylate is a useful research compound. Its molecular formula is C14H20N2O2 and its molecular weight is 248.32 g/mol. The purity is usually 95%.
BenchChem offers high-quality this compound suitable for many research applications. Different packaging options are available to accommodate customers' requirements. Please inquire for more information about this compound including the price, delivery time, and more detailed information at info@benchchem.com.
Scientific Research Applications
Synthesis and Crystal Structure
- "tert-Butyl 2-((phenylthio)carbonyl)pyrrolidine-1-carboxylate" was synthesized using iso-butoxycarbonyl chloride via a mixed anhydride method. Its crystal structure was determined, showing it crystallizes in the triclinic space group with specific cell parameters. The proline ring in the structure adopts an envelope conformation, and the structure displays intermolecular hydrogen bonds (Naveen et al., 2007).
Coupling Reactions
- "tert-Butylcarbonyl 1,2,3,6-tetrahydro-4-[(trifluoromethyl)sulfonyloxy]pyridine-1-carboxylate" was prepared and underwent palladium-catalyzed coupling reactions with various substituted arylboronic acids. This process produced a series of tert-butyl-4-aryl-1,2,3,6-tetrahydropyridine-1-carboxylates (Wustrow & Wise, 1991).
Asymmetric Synthesis
- An asymmetric synthesis method was described for N-tert-butyl disubstituted pyrrolidines via a nitrile anion cyclization strategy. This involved a five-step, chromatography-free synthesis from 2-chloro-1-(2,4-difluorophenyl)-ethanone, achieving a 71% overall yield. The key cyclization concomitantly formed the pyrrolidine ring with clean inversion of the C-4 center, yielding chiral pyrrolidine in high yield and enantiomeric excess (Chung et al., 2005).
Crystal Structure Analysis
- "tert-butyl {1(S)-[(3R,4S,5S)-5-benzyl-4-hydroxy-2-oxo-3-pyrrolidinyl]-2-phenylethyl} -carbamate" is an example of an all-cis trisubstituted pyrrolidin-2-one. Its crystal structure was analyzed, revealing intramolecular hydrogen bonding between the pyrrolidin-2-one carbonyl O atom and the N-H group of the L-phenylalanine fragment (Weber et al., 1995).
Mechanism of Action
Target of action
Pyrrolidine derivatives are often used in medicinal chemistry due to their ability to interact with various biological targets .
Mode of action
Without specific information, it’s difficult to say how this compound interacts with its targets. Generally, the pyrrolidine ring can contribute to the stereochemistry of the molecule, and its non-planarity can increase three-dimensional coverage, a phenomenon called "pseudorotation" .
Biochemical pathways
Again, without specific information, it’s hard to say which biochemical pathways this compound affects. Pyrrolidine derivatives can affect a wide range of pathways depending on their specific structure and targets .
Pharmacokinetics
The introduction of heteroatomic fragments in these molecules is a common strategy for modifying physicochemical parameters and obtaining the best adme/tox results for drug candidates .
Action environment
Environmental factors such as temperature, pH, and the presence of other molecules can influence the action, efficacy, and stability of a compound. For example, some compounds need to be stored under specific conditions to maintain their stability .
Properties
IUPAC Name |
tert-butyl 2-pyridin-4-ylpyrrolidine-1-carboxylate | |
---|---|---|
Source | PubChem | |
URL | https://pubchem.ncbi.nlm.nih.gov | |
Description | Data deposited in or computed by PubChem | |
InChI |
InChI=1S/C14H20N2O2/c1-14(2,3)18-13(17)16-10-4-5-12(16)11-6-8-15-9-7-11/h6-9,12H,4-5,10H2,1-3H3 | |
Source | PubChem | |
URL | https://pubchem.ncbi.nlm.nih.gov | |
Description | Data deposited in or computed by PubChem | |
InChI Key |
JERJWJYZAWNCDM-UHFFFAOYSA-N | |
Source | PubChem | |
URL | https://pubchem.ncbi.nlm.nih.gov | |
Description | Data deposited in or computed by PubChem | |
Canonical SMILES |
CC(C)(C)OC(=O)N1CCCC1C2=CC=NC=C2 | |
Source | PubChem | |
URL | https://pubchem.ncbi.nlm.nih.gov | |
Description | Data deposited in or computed by PubChem | |
Molecular Formula |
C14H20N2O2 | |
Source | PubChem | |
URL | https://pubchem.ncbi.nlm.nih.gov | |
Description | Data deposited in or computed by PubChem | |
Molecular Weight |
248.32 g/mol | |
Source | PubChem | |
URL | https://pubchem.ncbi.nlm.nih.gov | |
Description | Data deposited in or computed by PubChem | |
Retrosynthesis Analysis
AI-Powered Synthesis Planning: Our tool employs the Template_relevance Pistachio, Template_relevance Bkms_metabolic, Template_relevance Pistachio_ringbreaker, Template_relevance Reaxys, Template_relevance Reaxys_biocatalysis model, leveraging a vast database of chemical reactions to predict feasible synthetic routes.
One-Step Synthesis Focus: Specifically designed for one-step synthesis, it provides concise and direct routes for your target compounds, streamlining the synthesis process.
Accurate Predictions: Utilizing the extensive PISTACHIO, BKMS_METABOLIC, PISTACHIO_RINGBREAKER, REAXYS, REAXYS_BIOCATALYSIS database, our tool offers high-accuracy predictions, reflecting the latest in chemical research and data.
Strategy Settings
Precursor scoring | Relevance Heuristic |
---|---|
Min. plausibility | 0.01 |
Model | Template_relevance |
Template Set | Pistachio/Bkms_metabolic/Pistachio_ringbreaker/Reaxys/Reaxys_biocatalysis |
Top-N result to add to graph | 6 |
Feasible Synthetic Routes
Disclaimer and Information on In-Vitro Research Products
Please be aware that all articles and product information presented on BenchChem are intended solely for informational purposes. The products available for purchase on BenchChem are specifically designed for in-vitro studies, which are conducted outside of living organisms. In-vitro studies, derived from the Latin term "in glass," involve experiments performed in controlled laboratory settings using cells or tissues. It is important to note that these products are not categorized as medicines or drugs, and they have not received approval from the FDA for the prevention, treatment, or cure of any medical condition, ailment, or disease. We must emphasize that any form of bodily introduction of these products into humans or animals is strictly prohibited by law. It is essential to adhere to these guidelines to ensure compliance with legal and ethical standards in research and experimentation.