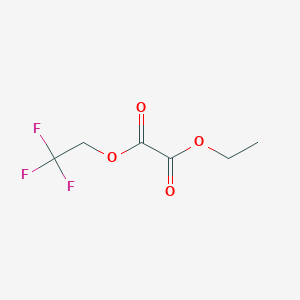
Ethyl 2,2,2-trifluoroethyl oxalate
Overview
Description
Ethyl 2,2,2-trifluoroethyl oxalate is a trifluoroethyl ester of oxalic acid. It is a colorless, odorless, and non-toxic liquid with a boiling point of 98°C and a melting point of -60°C . This compound is used in various applications, including organic synthesis, pharmaceuticals, and biochemistry .
Mechanism of Action
Target of Action
Ethyl 2,2,2-trifluoroethyl oxalate is primarily used in the field of energy storage, specifically in lithium-ion batteries and electrochemical capacitors . Its primary targets are the electrolytes in these devices, where it acts as a fluorinated additive .
Mode of Action
The compound interacts with its targets by enhancing their stability, particularly at high voltages . It increases the operating limits of electric double-layer type supercapacitors, suggesting its potential in enhancing energy storage and compatibility with lithium-ion battery cells .
Biochemical Pathways
The compound affects the biochemical pathways related to energy storage and transfer in lithium-ion batteries and electrochemical capacitors . By improving the stability of the electrolytes, it prevents extensive electrolyte oxidation, which is crucial for enhancing the longevity and efficiency of these devices .
Result of Action
The result of the compound’s action is significant improvement in self-discharge behavior and high-temperature cycle performance in lithium-ion batteries . In the context of electrochemical capacitors, it shows promise in increasing their operating limits .
Biochemical Analysis
Biochemical Properties
Ethyl 2,2,2-trifluoroethyl oxalate plays a significant role in biochemical reactions due to its unique chemical structure. It interacts with various enzymes, proteins, and other biomolecules. For instance, it has been studied as a fluorinated additive in electrochemical capacitor electrolytes, where it improves stability at high voltages . The compound’s interactions with biomolecules often involve the ester linkage (C-O-C) between the ethyl group and the oxalate, as well as reactions involving the carbonyl groups and the trifluoroethyl moiety.
Cellular Effects
This compound influences various cellular processes. It has been shown to affect cell function by interacting with cell signaling pathways, gene expression, and cellular metabolism. For example, in lithium-ion batteries, the compound’s stability prevents extensive electrolyte oxidation, which is crucial for enhancing the longevity and efficiency of the cells . This suggests that this compound may have similar stabilizing effects on cellular components in biological systems.
Molecular Mechanism
The molecular mechanism of this compound involves its binding interactions with biomolecules, enzyme inhibition or activation, and changes in gene expression. The compound’s ester linkage and oxalate group are key functional groups that participate in these interactions. For instance, the trifluoroethyl group can form hydrogen bonds with biomolecules, influencing their structure and function.
Temporal Effects in Laboratory Settings
In laboratory settings, the effects of this compound change over time. The compound is stable under high pressure and can form films that increase the flash point, enhancing safety Over time, its stability and low volatility make it a reliable reagent for long-term experiments
Dosage Effects in Animal Models
The effects of this compound vary with different dosages in animal models. At low doses, the compound may exhibit beneficial effects, such as stabilizing cellular components and enhancing metabolic processes. At high doses, it may cause toxic or adverse effects. Studies on lithium-ion batteries have shown that the compound’s stability prevents extensive oxidation, suggesting that it may have a threshold effect in biological systems as well .
Metabolic Pathways
This compound is involved in various metabolic pathways. It interacts with enzymes and cofactors, influencing metabolic flux and metabolite levels. The compound’s ester linkage can be susceptible to hydrolysis under acidic or basic conditions, yielding oxalic acid, ethanol, and trifluoroethanol. These metabolic products can further participate in biochemical reactions, affecting overall cellular metabolism.
Transport and Distribution
Within cells and tissues, this compound is transported and distributed through interactions with transporters and binding proteins. Its non-polar trifluoroethyl group may limit its solubility in water, affecting its localization and accumulation in specific cellular compartments. The compound’s ability to form films and increase the flash point suggests that it may accumulate in lipid-rich areas of cells and tissues .
Subcellular Localization
This compound’s subcellular localization is influenced by its chemical properties and interactions with biomolecules. The compound may be directed to specific compartments or organelles through targeting signals or post-translational modifications. Its ester linkage and oxalate group can interact with cellular components, affecting its activity and function
Preparation Methods
Ethyl 2,2,2-trifluoroethyl oxalate can be synthesized using a simple parallel synthesis approach. This method involves the use of mthis compound and bis 2,2,2-trifluoroethyl oxalate . The reaction conditions typically involve the use of hindered primary and secondary aliphatic amines, resulting in the formation of unsymmetrical N1, N2-substituted aliphatic oxamides . The products are obtained with a high overall success rate in moderate yields .
Chemical Reactions Analysis
Ethyl 2,2,2-trifluoroethyl oxalate undergoes various types of chemical reactions, including oxidation, reduction, and substitution . Common reagents used in these reactions include trifluoroacetic acid and trifluoroethoxy group . The major products formed from these reactions are trifluoroacetic acid and trifluoroethyl vinyl ether .
Scientific Research Applications
Ethyl 2,2,2-trifluoroethyl oxalate is used in a variety of scientific research applications. It is utilized in organic synthesis, pharmaceuticals, and biochemistry due to its stability and low volatility . In the field of energy storage, it is used as a fluorinated additive in electrochemical capacitor electrolytes, improving stability at high voltages . Additionally, it is employed in the development of non-flammable electrolytes for lithium-ion batteries, enhancing safety and compatibility with graphite anodes . The compound also shows promise in improving the performance of high-voltage cathodes in lithium-ion batteries .
Comparison with Similar Compounds
Ethyl 2,2,2-trifluoroethyl oxalate can be compared with other similar compounds, such as mthis compound and bis 2,2,2-trifluoroethyl oxalate . These compounds share similar chemical properties and are used in parallel synthesis approaches to produce unsymmetrical N1, N2-substituted aliphatic oxamides . this compound is unique due to its specific applications in energy storage and non-flammable electrolytes for lithium-ion batteries .
Properties
IUPAC Name |
1-O-ethyl 2-O-(2,2,2-trifluoroethyl) oxalate | |
---|---|---|
Source | PubChem | |
URL | https://pubchem.ncbi.nlm.nih.gov | |
Description | Data deposited in or computed by PubChem | |
InChI |
InChI=1S/C6H7F3O4/c1-2-12-4(10)5(11)13-3-6(7,8)9/h2-3H2,1H3 | |
Source | PubChem | |
URL | https://pubchem.ncbi.nlm.nih.gov | |
Description | Data deposited in or computed by PubChem | |
InChI Key |
KWTUHAQSICWITR-UHFFFAOYSA-N | |
Source | PubChem | |
URL | https://pubchem.ncbi.nlm.nih.gov | |
Description | Data deposited in or computed by PubChem | |
Canonical SMILES |
CCOC(=O)C(=O)OCC(F)(F)F | |
Source | PubChem | |
URL | https://pubchem.ncbi.nlm.nih.gov | |
Description | Data deposited in or computed by PubChem | |
Molecular Formula |
C6H7F3O4 | |
Source | PubChem | |
URL | https://pubchem.ncbi.nlm.nih.gov | |
Description | Data deposited in or computed by PubChem | |
Molecular Weight |
200.11 g/mol | |
Source | PubChem | |
URL | https://pubchem.ncbi.nlm.nih.gov | |
Description | Data deposited in or computed by PubChem | |
Retrosynthesis Analysis
AI-Powered Synthesis Planning: Our tool employs the Template_relevance Pistachio, Template_relevance Bkms_metabolic, Template_relevance Pistachio_ringbreaker, Template_relevance Reaxys, Template_relevance Reaxys_biocatalysis model, leveraging a vast database of chemical reactions to predict feasible synthetic routes.
One-Step Synthesis Focus: Specifically designed for one-step synthesis, it provides concise and direct routes for your target compounds, streamlining the synthesis process.
Accurate Predictions: Utilizing the extensive PISTACHIO, BKMS_METABOLIC, PISTACHIO_RINGBREAKER, REAXYS, REAXYS_BIOCATALYSIS database, our tool offers high-accuracy predictions, reflecting the latest in chemical research and data.
Strategy Settings
Precursor scoring | Relevance Heuristic |
---|---|
Min. plausibility | 0.01 |
Model | Template_relevance |
Template Set | Pistachio/Bkms_metabolic/Pistachio_ringbreaker/Reaxys/Reaxys_biocatalysis |
Top-N result to add to graph | 6 |
Feasible Synthetic Routes
Disclaimer and Information on In-Vitro Research Products
Please be aware that all articles and product information presented on BenchChem are intended solely for informational purposes. The products available for purchase on BenchChem are specifically designed for in-vitro studies, which are conducted outside of living organisms. In-vitro studies, derived from the Latin term "in glass," involve experiments performed in controlled laboratory settings using cells or tissues. It is important to note that these products are not categorized as medicines or drugs, and they have not received approval from the FDA for the prevention, treatment, or cure of any medical condition, ailment, or disease. We must emphasize that any form of bodily introduction of these products into humans or animals is strictly prohibited by law. It is essential to adhere to these guidelines to ensure compliance with legal and ethical standards in research and experimentation.