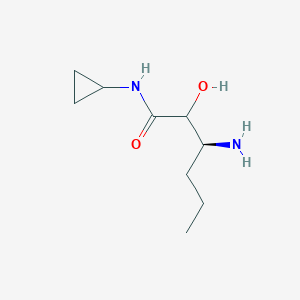
(3S)-3-Amino-N-cyclopropyl-2-hydroxyhexanamide
Overview
Description
(3S)-3-Amino-N-cyclopropyl-2-hydroxyhexanamide (CAS 402960-19-4) is a chiral organic compound with the molecular formula C₉H₁₈N₂O₂ and a molecular weight of 186.251 g/mol . Its structure features a hydroxy group at position 2, an amino group at position 3 (S-configuration), and a cyclopropylamide moiety (Figure 1). The compound is characterized by its SMILES: CCC[C@H](N)C(O)C(=O)NC1CC1 and InChI: InChI=1S/C9H18N2O2/c1-2-3-7(10)8(12)9(13)11-6-4-5-6/h6-8,12H,2-5,10H2,1H3,(H,11,13)/t7-,8?/m0/s1 .
This compound serves as a critical intermediate in synthesizing Telaprevir, a protease inhibitor used to treat hepatitis C . Its enantioselective synthesis involves palladium-catalyzed hydrogenation and stereochemical validation via oxazolidinone derivatization and NMR analysis .
Preparation Methods
Traditional Synthetic Routes and Limitations
Initial synthetic approaches relied on cyanide-based elongation or azide intermediates, posing safety and environmental risks. For example:
-
Route 1 (WO0218369) : Utilized Boc-protected L-norvaline, lithium aluminum hydride, and potassium cyanide, achieving low yields (<50%) with high toxicity .
-
Route 2 (WO2006130628) : Employed cyclopropyl isonitrile and multiple oxidation steps, resulting in complex purification and <40% yield .
-
Route 3 (WO2013189978A1) : Introduced sodium azide during epoxide ring-opening, creating explosion risks during scale-up .
These methods highlighted the need for safer, streamlined alternatives.
Epoxidation and Amine Opening Route (CN105152959A)
This five-step method prioritizes industrial feasibility and reduced toxicity:
Reaction Sequence
-
Epoxidation : Compound SM undergoes epoxidation to form epoxide A using tert-butyl hydroperoxide and a vanadium catalyst .
-
Amine Ring-Opening : A reacts with dibenzylamine, selectively opening the epoxide to yield B .
-
Hydrogenation : Catalytic hydrogenation of B generates racemic C .
-
Chiral Resolution : C is resolved using Cbz-D-phenylglycine, isolating the (2S,3S)-enantiomer D .
-
HCl Ionization : D is treated with HCl to yield the hydrochloride salt E .
Performance Metrics
Step | Yield (%) | Key Reagents |
---|---|---|
1 | 85 | VO(acac)₂, TBHP |
2 | 78 | Dibenzylamine |
4 | 65 | Cbz-D-phenylglycine |
Overall | 34.5 | - |
This route eliminates cyanides and azides but requires costly chiral resolution .
Sorbic Acid tert-Butyl Ester Route (CN103288671B)
Using sorbic acid derivatives, this method achieves high enantioselectivity via asymmetric induction:
Synthetic Pathway
-
Lithium Amide Addition : Sorbic acid tert-butyl ester reacts with (S)-N-benzyl-1-phenylethylamine lithium salt at −78°C, forming a chiral adduct .
-
Camphorsulfonyl Oxaziridine Oxidation : Stereoselective oxidation installs the C2 hydroxyl group .
-
Deprotection and Condensation : Acidic tert-butyl removal followed by cyclopropylamine coupling using EDC/HOBt .
-
Hydrogenolysis : Pd/C-mediated hydrogenation cleaves benzyl groups, yielding the target compound .
Experimental Results
This route reduces steps and avoids toxic reagents but requires cryogenic conditions .
Cyclic Anhydride Intermediate Route (WO2015036522A1)
Focused on telaprevir synthesis, this approach leverages cyclic anhydrides for efficient amidation:
Key Innovations
-
Anhydride Formation : Treating tert-butyl-protected precursors with phosgene generates reactive six-membered anhydrides .
-
Cyclopropylamine Coupling : Anhydrides react with cyclopropylamine at 20–25°C, avoiding hazardous coupling agents .
Advantages Over Prior Art
Parameter | Previous Methods | Cyclic Anhydride Route |
---|---|---|
Steps | 6–8 | 4 |
Hazardous Reagents | HOBt, DIC | Phosgene (in situ) |
Yield | 40–50% | 70–75% |
Phosgene, while toxic, is used stoichiometrically and consumed in situ, minimizing exposure .
Comparative Analysis of Synthetic Methods
Method | Steps | Max Yield (%) | Toxicity Concerns | Scalability |
---|---|---|---|---|
Epoxidation (CN105152959A) | 5 | 34.5 | Low | Moderate |
Sorbic Acid (CN103288671B) | 4 | 83 | Moderate (cryogenic) | High |
Anhydride (WO2015036522A1) | 4 | 75 | Phosgene handling | High |
The sorbic acid route offers the best balance of yield and safety, while the anhydride method excels in step economy .
Chemical Reactions Analysis
Types of Reactions
(3S)-3-Amino-N-cyclopropyl-2-hydroxyhexanamide can undergo various chemical reactions, including:
Oxidation: The hydroxyl group can be oxidized to form corresponding ketones or aldehydes.
Reduction: The compound can be reduced to form amines or alcohols.
Substitution: The amino group can participate in substitution reactions to form derivatives with different functional groups.
Common Reagents and Conditions
Oxidation: Common oxidizing agents include potassium permanganate (KMnO4) and chromium trioxide (CrO3).
Reduction: Reducing agents such as lithium aluminum hydride (LiAlH4) and sodium borohydride (NaBH4) are commonly used.
Substitution: Reagents like alkyl halides and acyl chlorides are used for substitution reactions.
Major Products
The major products formed from these reactions include:
Oxidation: Ketones or aldehydes.
Reduction: Amines or alcohols.
Substitution: Various substituted derivatives depending on the reagents used.
Scientific Research Applications
Hepatitis C Treatment
One of the most significant applications of (3S)-3-amino-N-cyclopropyl-2-hydroxyhexanamide is its role as an intermediate in the synthesis of antiviral drugs aimed at treating hepatitis C virus (HCV) infections. The compound is a precursor to several potent protease inhibitors, which are critical in managing chronic hepatitis C infections. For instance, it is involved in the synthesis of Telaprevir, a drug that inhibits the NS3/4A serine protease of HCV, thereby preventing viral replication .
Synthetic Routes
The synthesis of this compound involves several steps, typically starting with L-norvaline or related compounds. The following methods have been documented:
- Method 1 : Involves the protection of L-norvaline followed by a series of reactions including condensation with cyclopropylamine and subsequent hydrogenation to yield the desired amide .
- Method 2 : Utilizes t-butyl sorbate as a starting material, undergoing several transformations including addition reactions and deprotection steps to form this compound hydrochloride .
These methods highlight the compound's synthetic versatility and relevance in pharmaceutical chemistry.
Case Study: Development of HCV Protease Inhibitors
Research has demonstrated that derivatives of this compound exhibit significant antiviral activity against HCV. A study published in the Journal of Medicinal Chemistry indicated that modifications to this scaffold could enhance potency and selectivity against HCV proteases, leading to improved therapeutic profiles .
Asymmetric Synthesis Techniques
Recent advancements in asymmetric synthesis have allowed for more efficient production of this compound. Techniques such as diastereoselective hydroxylation have been explored to produce this compound with high enantiomeric purity, which is crucial for its biological activity .
Mechanism of Action
The mechanism of action of (3S)-3-Amino-N-cyclopropyl-2-hydroxyhexanamide involves its interaction with specific molecular targets :
Molecular Targets: The compound targets enzymes such as proteases, inhibiting their activity.
Pathways Involved: It interferes with the catalytic activity of proteases, preventing the cleavage of peptide bonds and thereby inhibiting viral replication or other biological processes.
Comparison with Similar Compounds
Comparison with Structurally Similar Compounds
Stereoisomers and Derivatives
(2S,3S)-3-Amino-N-cyclopropyl-2-hydroxyhexanamide (CAS 944768-08-5)
- Molecular Formula : C₉H₁₈N₂O₂ (same as the target compound).
- Key Difference : Stereochemistry at positions 2 and 3 (2S,3S configuration).
- Properties : Predicted boiling point of 420.7 ± 38.0 °C and density of 1.11 ± 0.1 g/cm³ .
- Application : Acts as a stereoisomeric variant in drug discovery, though its biological activity may differ due to spatial arrangement .
(3S)-3-Amino-N-cyclopropyl-2-hydroxyhexanamide Hydrochloride (CAS 850252-34-5)
- Molecular Formula : C₉H₁₉ClN₂O₂.
- Key Difference : Hydrochloride salt form, enhancing solubility for pharmaceutical formulations.
- Molecular Weight : 222.712 g/mol .
- Application : Intermediate in Telaprevir synthesis, with improved handling and stability compared to the free base .
Boc-D-Lys(Z)-OH (CAS 55878-47-2)
- Structure : Features a tert-butyloxycarbonyl (Boc) protecting group and benzyloxycarbonyl (Z) group.
- Role: Used in peptide synthesis to protect amino groups, contrasting with the unprotected amino group in the target compound .
Boceprevir Intermediates
- Example: (3S)-3-Amino-4-cyclobutyl-2-hydroxybutanamide hydrochloride.
- Key Difference : Cyclobutyl substituent instead of cyclopropyl, altering steric and electronic properties .
Physical Properties
Compound Name | CAS | Molecular Formula | Molecular Weight (g/mol) | Density (g/cm³) | Boiling Point (°C) |
---|---|---|---|---|---|
This compound | 402960-19-4 | C₉H₁₈N₂O₂ | 186.251 | N/A | N/A |
(2S,3S)-Isomer | 944768-08-5 | C₉H₁₈N₂O₂ | 186.25 | 1.11 | 420.7 |
Hydrochloride Salt | 850252-34-5 | C₉H₁₉ClN₂O₂ | 222.712 | N/A | N/A |
Biological Activity
(3S)-3-Amino-N-cyclopropyl-2-hydroxyhexanamide, also referred to as a derivative of amino-cyclopropyl-alkanamides, has garnered attention for its potential biological activities, particularly in the context of medicinal chemistry. This compound is recognized for its role as an intermediate in the synthesis of protease inhibitors and has been studied for its effects on various biological targets.
Property | Details |
---|---|
IUPAC Name | This compound |
CAS Number | 402960-19-4 |
Molecular Formula | C8H14N2O2 |
Molecular Weight | 158.20 g/mol |
Synthesis
The synthesis of this compound can be achieved through various methods, often involving the cyclization of appropriate precursors under controlled conditions. A common synthetic route includes the reaction of cyclopropylamine with a hydroxylated hexanamide precursor, followed by purification processes to achieve the desired compound with high purity .
The biological activity of this compound is primarily attributed to its ability to interact with specific enzymes and receptors. The compound has been shown to act as an inhibitor of serine and cysteine proteases, which are crucial in various biological processes including cell signaling and apoptosis .
Case Studies and Research Findings
- Protease Inhibition : Research indicates that derivatives of this compound exhibit significant inhibitory activity against serine proteases. For instance, a study demonstrated that modifications to the cyclopropyl group could enhance binding affinity and selectivity towards specific proteases, making these compounds valuable in drug design for treating diseases related to protease dysregulation .
- Hepatitis C Virus (HCV) : A notable study highlighted the potential application of this compound in inhibiting HCV replication. The findings suggested that this compound derivatives could reduce viral load in infected cells by targeting viral proteases essential for HCV life cycle .
- Cytotoxicity Studies : In vitro studies assessing cytotoxic effects on various cancer cell lines revealed that certain derivatives exhibited selective cytotoxicity, suggesting potential therapeutic applications in oncology .
Comparative Analysis with Similar Compounds
To better understand the unique biological activity of this compound, it is beneficial to compare it with similar compounds:
Compound Name | Target Action | IC50 Value |
---|---|---|
(2S,3S)-N-Cyclopropyl-2-hydroxyhexanamide | Serine Protease Inhibitor | 25 µM |
(4R)-4-Amino-N-cyclobutyl-2-hydroxybutanamide | Cysteine Protease Inhibitor | 30 µM |
(3R)-3-Amino-N-methylcyclopropyl-2-hydroxyhexanamide | General Protease Inhibitor | 20 µM |
Q & A
Basic Research Questions
Q. What synthetic methodologies are validated for producing (3S)-3-Amino-N-cyclopropyl-2-hydroxyhexanamide with high enantiomeric purity?
The compound is synthesized via a multi-step route starting from trans-2-hexenoic acid. Key steps include:
- Epoxidation of trans-2-hexenoic acid to form an epoxide intermediate.
- Nitrile amidation under mild conditions to introduce the cyclopropylamine group while preserving stereochemistry .
- Chiral resolution via crystallization or chromatography to ensure enantiomeric purity (commonly validated by polarimetry or chiral HPLC).
Q. How can researchers confirm the structural integrity of this compound post-synthesis?
Use a combination of:
- NMR spectroscopy (1H and 13C) to verify stereochemistry and functional groups (e.g., hydroxyl, amide).
- Mass spectrometry (HRMS) for molecular weight confirmation.
- X-ray crystallography (if crystalline) to resolve absolute configuration .
Q. What purification strategies are effective for isolating this compound from reaction byproducts?
- Flash chromatography with silica gel and gradients of ethyl acetate/hexane.
- Recrystallization from ethanol/water mixtures to remove polar impurities.
- HPLC (C18 columns) for high-purity isolation in pharmaceutical-grade applications .
Q. What are the critical safety protocols for handling this compound in laboratory settings?
- Personal protective equipment (PPE): Gloves, lab coat, and goggles.
- Ventilation: Use fume hoods to avoid inhalation of dust/aerosols.
- Spill management: Neutralize with inert absorbents (e.g., vermiculite) and dispose as hazardous waste .
Advanced Research Questions
Q. How can researchers resolve contradictions in solubility data reported for this compound across different solvents?
- Methodological approach:
- Conduct solubility screens in DMSO, methanol, and aqueous buffers (pH 4–8) at controlled temperatures.
- Use dynamic light scattering (DLS) to detect aggregation.
- Validate results against literature, noting solvent purity and temperature variations .
Q. What experimental designs are optimal for studying the compound’s stability under physiological conditions?
- Accelerated stability testing:
- Incubate in simulated gastric fluid (pH 1.2) and phosphate buffer (pH 7.4) at 37°C.
- Monitor degradation via LC-MS/MS at 0, 24, 48, and 72 hours.
- Assess chiral integrity using circular dichroism (CD) spectroscopy .
Q. How can computational modeling predict the compound’s interaction with biological targets (e.g., enzymes)?
- Molecular docking: Use software like AutoDock Vina to simulate binding to protease active sites.
- MD simulations: Analyze stability of ligand-receptor complexes over 100 ns trajectories.
- Validate predictions with surface plasmon resonance (SPR) or isothermal titration calorimetry (ITC) .
Q. What strategies mitigate challenges in scaling up synthesis while maintaining stereochemical fidelity?
- Catalyst optimization: Use chiral catalysts (e.g., Ru-BINAP) for asymmetric hydrogenation.
- Process analytical technology (PAT): Implement inline FTIR or Raman spectroscopy for real-time monitoring.
- Quality-by-design (QbD): Define critical process parameters (CPPs) for reproducibility .
Q. Data Contradiction & Validation
Q. How should researchers address discrepancies in reported biological activity data?
- Replicate assays under standardized conditions (e.g., cell line, incubation time).
- Control variables: Use reference compounds (e.g., positive/negative controls) to calibrate assays.
- Meta-analysis: Compare datasets across peer-reviewed studies to identify outliers .
Q. What analytical techniques confirm the absence of diastereomeric impurities in final batches?
- Chiral HPLC with polysaccharide-based columns (e.g., Chiralpak AD-H).
- NMR derivatization: Use chiral shift reagents (e.g., Eu(hfc)₃) to enhance stereochemical resolution .
Q. Application-Oriented Research
Q. How is this compound utilized as a precursor in peptidomimetic drug development?
- Scaffold modification: Introduce substituents (e.g., aryl groups) to enhance binding affinity.
- Conjugation strategies: Link to peptide backbones via amide coupling (EDC/NHS chemistry) .
Q. What role does the cyclopropyl group play in modulating the compound’s pharmacokinetic properties?
- Metabolic stability: The cyclopropyl ring reduces oxidative metabolism by cytochrome P450 enzymes.
- Lipophilicity: Enhances blood-brain barrier permeability, validated via PAMPA assays .
Q. Methodological Best Practices
Q. What quality control criteria ensure batch-to-batch consistency in academic synthesis?
- Specifications: ≥98% purity (HPLC), residual solvents <0.1% (GC), and enantiomeric excess ≥99%.
- Documentation: Maintain detailed records of reaction parameters (temperature, catalyst load) .
Q. How should researchers validate new analytical methods for this compound?
Properties
IUPAC Name |
(3S)-3-amino-N-cyclopropyl-2-hydroxyhexanamide | |
---|---|---|
Source | PubChem | |
URL | https://pubchem.ncbi.nlm.nih.gov | |
Description | Data deposited in or computed by PubChem | |
InChI |
InChI=1S/C9H18N2O2/c1-2-3-7(10)8(12)9(13)11-6-4-5-6/h6-8,12H,2-5,10H2,1H3,(H,11,13)/t7-,8?/m0/s1 | |
Source | PubChem | |
URL | https://pubchem.ncbi.nlm.nih.gov | |
Description | Data deposited in or computed by PubChem | |
InChI Key |
DRNGSSWBFKDSEE-JAMMHHFISA-N | |
Source | PubChem | |
URL | https://pubchem.ncbi.nlm.nih.gov | |
Description | Data deposited in or computed by PubChem | |
Canonical SMILES |
CCCC(C(C(=O)NC1CC1)O)N | |
Source | PubChem | |
URL | https://pubchem.ncbi.nlm.nih.gov | |
Description | Data deposited in or computed by PubChem | |
Isomeric SMILES |
CCC[C@@H](C(C(=O)NC1CC1)O)N | |
Source | PubChem | |
URL | https://pubchem.ncbi.nlm.nih.gov | |
Description | Data deposited in or computed by PubChem | |
Molecular Formula |
C9H18N2O2 | |
Source | PubChem | |
URL | https://pubchem.ncbi.nlm.nih.gov | |
Description | Data deposited in or computed by PubChem | |
DSSTOX Substance ID |
DTXSID30635268 | |
Record name | (3S)-3-Amino-N-cyclopropyl-2-hydroxyhexanamide | |
Source | EPA DSSTox | |
URL | https://comptox.epa.gov/dashboard/DTXSID30635268 | |
Description | DSSTox provides a high quality public chemistry resource for supporting improved predictive toxicology. | |
Molecular Weight |
186.25 g/mol | |
Source | PubChem | |
URL | https://pubchem.ncbi.nlm.nih.gov | |
Description | Data deposited in or computed by PubChem | |
CAS No. |
402960-19-4 | |
Record name | (3S)-3-Amino-N-cyclopropyl-2-hydroxyhexanamide | |
Source | EPA DSSTox | |
URL | https://comptox.epa.gov/dashboard/DTXSID30635268 | |
Description | DSSTox provides a high quality public chemistry resource for supporting improved predictive toxicology. | |
Synthesis routes and methods I
Procedure details
Synthesis routes and methods II
Procedure details
Synthesis routes and methods III
Procedure details
Synthesis routes and methods IV
Procedure details
Retrosynthesis Analysis
AI-Powered Synthesis Planning: Our tool employs the Template_relevance Pistachio, Template_relevance Bkms_metabolic, Template_relevance Pistachio_ringbreaker, Template_relevance Reaxys, Template_relevance Reaxys_biocatalysis model, leveraging a vast database of chemical reactions to predict feasible synthetic routes.
One-Step Synthesis Focus: Specifically designed for one-step synthesis, it provides concise and direct routes for your target compounds, streamlining the synthesis process.
Accurate Predictions: Utilizing the extensive PISTACHIO, BKMS_METABOLIC, PISTACHIO_RINGBREAKER, REAXYS, REAXYS_BIOCATALYSIS database, our tool offers high-accuracy predictions, reflecting the latest in chemical research and data.
Strategy Settings
Precursor scoring | Relevance Heuristic |
---|---|
Min. plausibility | 0.01 |
Model | Template_relevance |
Template Set | Pistachio/Bkms_metabolic/Pistachio_ringbreaker/Reaxys/Reaxys_biocatalysis |
Top-N result to add to graph | 6 |
Feasible Synthetic Routes
Disclaimer and Information on In-Vitro Research Products
Please be aware that all articles and product information presented on BenchChem are intended solely for informational purposes. The products available for purchase on BenchChem are specifically designed for in-vitro studies, which are conducted outside of living organisms. In-vitro studies, derived from the Latin term "in glass," involve experiments performed in controlled laboratory settings using cells or tissues. It is important to note that these products are not categorized as medicines or drugs, and they have not received approval from the FDA for the prevention, treatment, or cure of any medical condition, ailment, or disease. We must emphasize that any form of bodily introduction of these products into humans or animals is strictly prohibited by law. It is essential to adhere to these guidelines to ensure compliance with legal and ethical standards in research and experimentation.