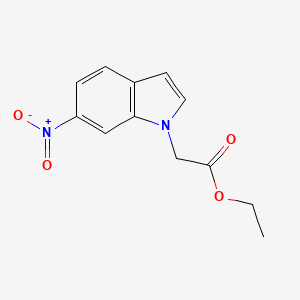
Ethyl 2-(6-nitro-1H-indol-1-yl)acetate
Overview
Description
Ethyl 2-(6-nitro-1H-indol-1-yl)acetate is a chemical compound with the linear formula C12H12N2O4 . It is a unique chemical provided to early discovery researchers . The compound is a pale-yellow to yellow-brown solid .
Molecular Structure Analysis
The molecular structure of this compound is represented by the linear formula C12H12N2O4 . The InChI code for the compound is 1S/C12H12N2O4/c1-2-18-12(15)8-13-6-5-9-3-4-10(14(16)17)7-11(9)13/h3-7H,2,8H2,1H3 .Physical And Chemical Properties Analysis
This compound is a pale-yellow to yellow-brown solid . It has a molecular weight of 248.24 . The compound is stored at room temperature .Scientific Research Applications
1. Synthesis and Characterization
Ethyl 2-[2-(4-nitrobenzoyl)-1H-indol-3-yl]acetate, closely related to Ethyl 2-(6-nitro-1H-indol-1-yl)acetate, was synthesized using aza-alkylation/intramolecular Michael cascade reaction and characterized using NMR, IR, and mass spectral data, showcasing its potential in chemical synthesis and analysis (Choi & Kim, 2017).
2. Novel Functionalized Indoles
Research on 1,3-disubstituted indole derivatives, including those related to this compound, led to the discovery of novel functionalized 2-hydroxyindolenines, indicating applications in the development of new chemical entities (Morales-Ríos et al., 1993).
3. Synthesis of Tryptamines and Carbolines
Ethyl 2-(3-indolyl)-3-nitroalkanoates, closely related compounds, are central intermediates in synthesizing tryptamines and carboline alkaloids, highlighting the compound's significance in medicinal chemistry (Ballini et al., 2008).
4. Crystallographic Studies
The crystal structure of a similar compound, (Z)-ethyl 3-[2-(5-methyl-7-nitro-1H-indole-2-carbonyl)hydrazinylidene]butanoate, has been determined, providing insights into the structural aspects of similar nitro-indole derivatives (Errossafi et al., 2015).
5. Synthesis of Novel Compounds
Ethyl 2-(2-(1H-indol-3-yl)benzo[d]thiazol-3(2H)-yl)-2-cyanoacetate, a compound structurally related to this compound, was synthesized, indicating its utility in creating new chemical entities for potential applications in drug development (Nassiri & Milani, 2020).
6. Corrosion Inhibition
Studies on 5-Nitro isatin derivatives, including this compound, have shown their potential use as corrosion inhibitors, adding another dimension to their application in material science (Ahmed, 2018).
Safety and Hazards
properties
IUPAC Name |
ethyl 2-(6-nitroindol-1-yl)acetate | |
---|---|---|
Source | PubChem | |
URL | https://pubchem.ncbi.nlm.nih.gov | |
Description | Data deposited in or computed by PubChem | |
InChI |
InChI=1S/C12H12N2O4/c1-2-18-12(15)8-13-6-5-9-3-4-10(14(16)17)7-11(9)13/h3-7H,2,8H2,1H3 | |
Source | PubChem | |
URL | https://pubchem.ncbi.nlm.nih.gov | |
Description | Data deposited in or computed by PubChem | |
InChI Key |
VGDUQCDAGWCFCT-UHFFFAOYSA-N | |
Source | PubChem | |
URL | https://pubchem.ncbi.nlm.nih.gov | |
Description | Data deposited in or computed by PubChem | |
Canonical SMILES |
CCOC(=O)CN1C=CC2=C1C=C(C=C2)[N+](=O)[O-] | |
Source | PubChem | |
URL | https://pubchem.ncbi.nlm.nih.gov | |
Description | Data deposited in or computed by PubChem | |
Molecular Formula |
C12H12N2O4 | |
Source | PubChem | |
URL | https://pubchem.ncbi.nlm.nih.gov | |
Description | Data deposited in or computed by PubChem | |
Molecular Weight |
248.23 g/mol | |
Source | PubChem | |
URL | https://pubchem.ncbi.nlm.nih.gov | |
Description | Data deposited in or computed by PubChem | |
Synthesis routes and methods
Procedure details
Retrosynthesis Analysis
AI-Powered Synthesis Planning: Our tool employs the Template_relevance Pistachio, Template_relevance Bkms_metabolic, Template_relevance Pistachio_ringbreaker, Template_relevance Reaxys, Template_relevance Reaxys_biocatalysis model, leveraging a vast database of chemical reactions to predict feasible synthetic routes.
One-Step Synthesis Focus: Specifically designed for one-step synthesis, it provides concise and direct routes for your target compounds, streamlining the synthesis process.
Accurate Predictions: Utilizing the extensive PISTACHIO, BKMS_METABOLIC, PISTACHIO_RINGBREAKER, REAXYS, REAXYS_BIOCATALYSIS database, our tool offers high-accuracy predictions, reflecting the latest in chemical research and data.
Strategy Settings
Precursor scoring | Relevance Heuristic |
---|---|
Min. plausibility | 0.01 |
Model | Template_relevance |
Template Set | Pistachio/Bkms_metabolic/Pistachio_ringbreaker/Reaxys/Reaxys_biocatalysis |
Top-N result to add to graph | 6 |
Feasible Synthetic Routes
Disclaimer and Information on In-Vitro Research Products
Please be aware that all articles and product information presented on BenchChem are intended solely for informational purposes. The products available for purchase on BenchChem are specifically designed for in-vitro studies, which are conducted outside of living organisms. In-vitro studies, derived from the Latin term "in glass," involve experiments performed in controlled laboratory settings using cells or tissues. It is important to note that these products are not categorized as medicines or drugs, and they have not received approval from the FDA for the prevention, treatment, or cure of any medical condition, ailment, or disease. We must emphasize that any form of bodily introduction of these products into humans or animals is strictly prohibited by law. It is essential to adhere to these guidelines to ensure compliance with legal and ethical standards in research and experimentation.