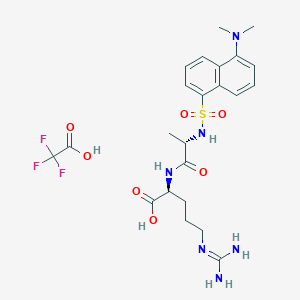
Dansyl-Ala-Arg-OH Trifluoroacetate
Overview
Description
Dansyl-Ala-Arg-OH Trifluoroacetate is a fluorescent substrate for carboxypeptidase M . The substrate and the cleavage product Dansyl-Ala-OH are equally fluorescent (λex = 340 nm; λem = 495 nm). The product has to be extracted with chloroform, and the uncleaved substrate remains in the aqueous phase (at acidic pH) .
Synthesis Analysis
Trifluoroacetic acid (TFA) is commonly used in the manufacturing process to release synthesized peptides from solid-phase resins . TFA or acetate is also used during reversed-phase HPLC purification of peptides .Molecular Structure Analysis
The molecular formula of Dansyl-Ala-Arg-OH Trifluoroacetate is C23H31F3N6O7S. Its molecular weight is 592.6 g/mol.Chemical Reactions Analysis
The reagent 1-dimethylaminonaphthalene-5-sulfonyl chloride (dansyl chloride, DNS-Cl) reacts with the free amino groups of peptides and proteins . Total acid hydrolysis of the substituted peptide or protein yields a mixture of free amino acids plus the dansyl derivative of the N-terminal amino acid .Physical And Chemical Properties Analysis
Dansyl-Ala-Arg-OH Trifluoroacetate is a fluorescent substrate for carboxypeptidase M . It has a molecular weight of 478.57 and a molecular formula of C21H30N6O5S .Scientific Research Applications
Protein Binding
Dansyl-Ala-Arg-OH Trifluoroacetate is used in laboratory procedures for binding enzymes or coupling peptides to carrier proteins. This process is crucial for developing relationships between proteins and other cellular components .
Chromatography
In chromatography, particularly High-Performance Liquid Chromatography (HPLC) , this compound is used during the purification of peptides. Trifluoroacetic acid (TFA), a component of Dansyl-Ala-Arg-OH Trifluoroacetate, is commonly used to release synthesized peptides from solid-phase resins .
Mass Spectrometry
Dansyl-Ala-Arg-OH Trifluoroacetate aids in sample manipulation during mass spectrometry by fulfilling essential laboratory functions and ensuring efficient and effective applications .
Amino Acid Quantification
The compound is involved in the quantification of amino acids in biological samples, which is a critical tool for studying metabolism. Dansylation, a process involving dansyl chloride, allows for the targeted quantification of amino acids .
Fluorescent Sensing
It serves as a fluorescent substrate for carboxypeptidase M, where both the substrate and cleavage product are equally fluorescent, facilitating the detection of specific reactions .
Pharmaceutical Manufacturing
Trifluoroacetic acid (TFA), part of this compound, is utilized in the manufacturing process of pharmaceuticals to aid in peptide synthesis and purification .
Mechanism of Action
Target of Action
Dansyl-Ala-Arg-OH Trifluoroacetate is primarily targeted towards carboxypeptidase M . Carboxypeptidase M is an enzyme that plays a crucial role in the metabolism of proteins and peptides, and is involved in various biological processes.
Mode of Action
Dansyl-Ala-Arg-OH Trifluoroacetate acts as a fluorescent substrate for carboxypeptidase M . The compound undergoes a reaction with free amines, yielding dansylated reaction products . This reaction is facilitated by dansyl chloride, which is incubated with amino acid standards or samples at room temperature in a sodium carbonate buffer for 60 minutes .
Biochemical Pathways
The biochemical pathway involved in the action of Dansyl-Ala-Arg-OH Trifluoroacetate is the metabolism of proteins and peptides. The compound, acting as a substrate, participates in the enzymatic reaction catalyzed by carboxypeptidase M . The resulting dansylated products are well-retained on reverse-phase columns .
Pharmacokinetics
The compound’s interaction with carboxypeptidase m and its subsequent reactions suggest that it may be metabolized and excreted following its enzymatic conversion .
Result of Action
The result of the action of Dansyl-Ala-Arg-OH Trifluoroacetate is the production of dansylated reaction products . These products are fluorescent, allowing for the quantification of amino acids in biological samples, which is a critical tool for studying metabolism .
Action Environment
The action of Dansyl-Ala-Arg-OH Trifluoroacetate is influenced by the pH of the environment. The uncleaved substrate remains in the aqueous phase at acidic pH . Additionally, the fluorescent properties of the substrate and the cleavage product (Dansyl-Ala-OH) are equally fluorescent, and the product has to be extracted with chloroform .
Safety and Hazards
properties
IUPAC Name |
(2S)-5-(diaminomethylideneamino)-2-[[(2S)-2-[[5-(dimethylamino)naphthalen-1-yl]sulfonylamino]propanoyl]amino]pentanoic acid;2,2,2-trifluoroacetic acid | |
---|---|---|
Source | PubChem | |
URL | https://pubchem.ncbi.nlm.nih.gov | |
Description | Data deposited in or computed by PubChem | |
InChI |
InChI=1S/C21H30N6O5S.C2HF3O2/c1-13(19(28)25-16(20(29)30)9-6-12-24-21(22)23)26-33(31,32)18-11-5-7-14-15(18)8-4-10-17(14)27(2)3;3-2(4,5)1(6)7/h4-5,7-8,10-11,13,16,26H,6,9,12H2,1-3H3,(H,25,28)(H,29,30)(H4,22,23,24);(H,6,7)/t13-,16-;/m0./s1 | |
Source | PubChem | |
URL | https://pubchem.ncbi.nlm.nih.gov | |
Description | Data deposited in or computed by PubChem | |
InChI Key |
LVOTWRDWOWKVRZ-LINSIKMZSA-N | |
Source | PubChem | |
URL | https://pubchem.ncbi.nlm.nih.gov | |
Description | Data deposited in or computed by PubChem | |
Canonical SMILES |
CC(C(=O)NC(CCCN=C(N)N)C(=O)O)NS(=O)(=O)C1=CC=CC2=C1C=CC=C2N(C)C.C(=O)(C(F)(F)F)O | |
Source | PubChem | |
URL | https://pubchem.ncbi.nlm.nih.gov | |
Description | Data deposited in or computed by PubChem | |
Isomeric SMILES |
C[C@@H](C(=O)N[C@@H](CCCN=C(N)N)C(=O)O)NS(=O)(=O)C1=CC=CC2=C1C=CC=C2N(C)C.C(=O)(C(F)(F)F)O | |
Source | PubChem | |
URL | https://pubchem.ncbi.nlm.nih.gov | |
Description | Data deposited in or computed by PubChem | |
Molecular Formula |
C23H31F3N6O7S | |
Source | PubChem | |
URL | https://pubchem.ncbi.nlm.nih.gov | |
Description | Data deposited in or computed by PubChem | |
Molecular Weight |
592.6 g/mol | |
Source | PubChem | |
URL | https://pubchem.ncbi.nlm.nih.gov | |
Description | Data deposited in or computed by PubChem | |
Product Name |
Dansyl-Ala-Arg-OH Trifluoroacetate |
Retrosynthesis Analysis
AI-Powered Synthesis Planning: Our tool employs the Template_relevance Pistachio, Template_relevance Bkms_metabolic, Template_relevance Pistachio_ringbreaker, Template_relevance Reaxys, Template_relevance Reaxys_biocatalysis model, leveraging a vast database of chemical reactions to predict feasible synthetic routes.
One-Step Synthesis Focus: Specifically designed for one-step synthesis, it provides concise and direct routes for your target compounds, streamlining the synthesis process.
Accurate Predictions: Utilizing the extensive PISTACHIO, BKMS_METABOLIC, PISTACHIO_RINGBREAKER, REAXYS, REAXYS_BIOCATALYSIS database, our tool offers high-accuracy predictions, reflecting the latest in chemical research and data.
Strategy Settings
Precursor scoring | Relevance Heuristic |
---|---|
Min. plausibility | 0.01 |
Model | Template_relevance |
Template Set | Pistachio/Bkms_metabolic/Pistachio_ringbreaker/Reaxys/Reaxys_biocatalysis |
Top-N result to add to graph | 6 |
Feasible Synthetic Routes
Disclaimer and Information on In-Vitro Research Products
Please be aware that all articles and product information presented on BenchChem are intended solely for informational purposes. The products available for purchase on BenchChem are specifically designed for in-vitro studies, which are conducted outside of living organisms. In-vitro studies, derived from the Latin term "in glass," involve experiments performed in controlled laboratory settings using cells or tissues. It is important to note that these products are not categorized as medicines or drugs, and they have not received approval from the FDA for the prevention, treatment, or cure of any medical condition, ailment, or disease. We must emphasize that any form of bodily introduction of these products into humans or animals is strictly prohibited by law. It is essential to adhere to these guidelines to ensure compliance with legal and ethical standards in research and experimentation.