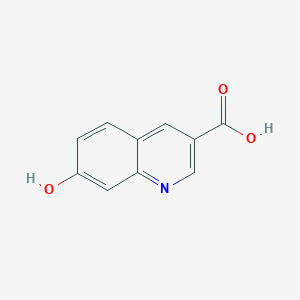
7-Hydroxyquinoline-3-carboxylic acid
Overview
Description
Preparation Methods
Direct Hydroxylation of Quinoline Derivatives
One of the classical approaches to synthesize hydroxyquinoline derivatives involves the hydroxylation of halogenated quinoline precursors.
Example Method: Hydrothermal Hydroxylation of 7-Bromoquinoline
Procedure:
In a hydrothermal synthesis reactor, sodium hydroxide (3 mmol) is dissolved in water (5 mL). 7-Bromoquinoline (0.5 mmol), cuprous oxide (0.05 mmol), and white hardwood alcohol (0.05 mmol) are added. The mixture is stirred at 130 °C for 6 hours. After cooling, pH is adjusted to 8 with dilute hydrochloric acid, and the product is extracted with ethyl acetate. Purification by column chromatography yields 7-hydroxyquinoline.Yield: 78%
- Key Reagents: Cuprous oxide as catalyst, sodium hydroxide as base
- Reaction Conditions: 130 °C, 6 hours, hydrothermal environment
This method, while efficient for 7-hydroxyquinoline, can be adapted for carboxylic acid derivatives by starting with appropriately substituted quinoline precursors.
Multi-Step Synthesis via Bromoisatin and Pyruvic Acid
A more elaborate but scalable and cost-effective method involves starting from 6-bromoisatin and pyruvic acid to build the quinoline core with the desired substitution pattern.
Step | Description | Conditions | Yield |
---|---|---|---|
1 | Synthesis of 7-bromoquinoline-2,4-carboxylic acid via rearrangement of 6-bromoisatin and pyruvic acid | Mix 6-bromoisatin (4 g, 17.9 mmol), 15% NaOH solution (24 mL), and pyruvic acid (2.7 g, 31.2 mmol); heat at 100 °C for 3 hours; cool to 0 °C; dilute with water and HCl; filter and dry | 55.3% (2.9 g) |
2 | Conversion to 7-bromoquinoline-4-carboxylic acid by elimination reaction | Add 7-bromoquinoline-2,4-carboxylic acid (4 g, 13.5 mmol) to nitrobenzene (35 mL) at 200 °C; reflux at 210 °C for 45 min; filter and purify | 79.3% (2.7 g) |
3 | Esterification to methyl ester (not detailed here) | Typically involves methanol and acid/base catalysis | Not specified |
4 | Amino protection and deprotection steps | Use of tert-butyloxycarbonyl (Boc) protection and hydrochloric acid for deprotection | Not specified |
5 | Diazotization and hydrolysis to 7-hydroxyquinoline-4-carboxylic acid | Diazotization in concentrated sulfuric acid; hydrolysis with sodium hydroxide | High total yield reported |
- Advantages:
- Uses inexpensive and readily available starting materials
- Avoids highly toxic reagents and expensive catalysts like palladium
- Suitable for large-scale production due to simple operations and high overall yield
- Process amenable to amplification and industrial application
This method is particularly notable for its avoidance of palladium catalysts and costly intermediates, making it economically favorable.
One-Pot Consecutive Synthesis in Aqueous Medium
For related hydroxyquinoline derivatives, a one-pot synthesis approach has been demonstrated, highlighting green chemistry principles.
- Starting Materials: 2,4-Dihydroxybenzaldehyde and malononitrile
- Procedure: Consecutive reactions in water, controlling pH to isolate product without organic solvents
- Outcome: Efficient synthesis of 7-hydroxy-3-carboxycoumarin, a structural analog relevant to quinoline chemistry
Though this method targets coumarin derivatives, it exemplifies the potential for environmentally friendly aqueous syntheses applicable to hydroxyquinoline carboxylic acids.
Comparative Summary Table of Preparation Methods
Preparation Method | Starting Materials | Key Steps | Reaction Conditions | Yield (%) | Advantages | Limitations |
---|---|---|---|---|---|---|
Hydrothermal hydroxylation of 7-bromoquinoline | 7-Bromoquinoline, NaOH, Cu2O | Hydroxylation at 130 °C, 6 h | 130 °C, 6 h, hydrothermal | 78 | Simple, moderate yield | Requires catalyst, purification needed |
Multi-step synthesis from 6-bromoisatin | 6-Bromoisatin, pyruvic acid, nitrobenzene | Rearrangement, elimination, esterification, diazotization, hydrolysis | 100–210 °C, various steps | >50 overall | Cost-effective, scalable, no Pd catalyst | Multi-step, longer synthesis time |
One-pot aqueous synthesis (analogous) | 2,4-Dihydroxybenzaldehyde, malononitrile | Consecutive reactions in water | Ambient to mild heating | Not specified | Green chemistry, solvent-free isolation | Target compound differs, adaptation needed |
Chemical Reactions Analysis
Types of Reactions: 7-Hydroxyquinoline-3-carboxylic acid undergoes various chemical reactions, including:
Oxidation: The hydroxyl group can be oxidized to form quinone derivatives.
Reduction: The carboxylic acid group can be reduced to form alcohol derivatives.
Common Reagents and Conditions:
Oxidation: Common oxidizing agents include potassium permanganate and chromium trioxide.
Reduction: Reducing agents such as lithium aluminum hydride and sodium borohydride are often used.
Substitution: Electrophilic reagents like halogens, nitrating agents, and sulfonating agents are employed under acidic or basic conditions.
Major Products: The major products formed from these reactions include quinone derivatives, alcohol derivatives, and various substituted quinoline compounds .
Scientific Research Applications
Medicinal Chemistry
7-Hydroxyquinoline-3-carboxylic acid has shown potential in medicinal chemistry due to its biological activities.
Antimicrobial Activity
Research indicates that derivatives of 7-hydroxyquinoline exhibit significant antimicrobial properties. A study by Bohn et al. (2009) demonstrated that certain derivatives could inhibit the growth of pathogenic bacteria and fungi, making them candidates for developing new antimicrobial agents .
Anticancer Properties
The compound has also been investigated for its anticancer effects. In vitro studies have shown that it can induce apoptosis in cancer cells through mechanisms involving oxidative stress and mitochondrial dysfunction .
Coordination Chemistry
The ability of this compound to form chelates with metal ions has been extensively studied.
Metal Ion Chelation
This compound acts as a bidentate ligand, coordinating with various metal ions such as iron and copper. The resulting complexes have applications in catalysis and as potential therapeutic agents for metal overload conditions .
Metal Ion | Complex Stability | Application |
---|---|---|
Fe(III) | High | Antioxidant properties |
Cu(II) | Moderate | Catalysis in organic reactions |
Analytical Chemistry
This compound is utilized in analytical methods for detecting metal ions.
Fluorescent Sensor Development
The compound's fluorescence properties make it suitable for developing sensors to detect trace levels of metal ions in environmental samples. Its sensitivity and selectivity are crucial for monitoring pollution levels .
Material Science
In material science, this compound is used to synthesize various functional materials.
Polymerization
It can act as a monomer in the synthesis of polymers with specific functionalities, such as enhanced thermal stability and improved mechanical properties. Research has explored its use in creating polymeric films that exhibit antimicrobial properties .
Case Study 1: Antimicrobial Efficacy
A study published in Organic & Biomolecular Chemistry evaluated the antimicrobial efficacy of several derivatives of this compound against Escherichia coli and Staphylococcus aureus. Results showed a minimum inhibitory concentration (MIC) as low as 32 µg/mL, indicating strong potential as an antimicrobial agent .
Case Study 2: Metal Ion Detection
In a project focused on environmental monitoring, researchers developed a fluorescent sensor based on this compound to detect lead ions in water samples. The sensor demonstrated a detection limit of 0.5 ppb, showcasing its effectiveness for real-time monitoring applications .
Mechanism of Action
The mechanism of action of 7-Hydroxyquinoline-3-carboxylic acid involves its interaction with various molecular targets:
Molecular Targets: The compound can bind to metal ions, forming stable complexes that inhibit the activity of metalloenzymes. It also interacts with nucleic acids and proteins, affecting their function.
Pathways Involved: The inhibition of metalloenzymes can disrupt essential biological processes, leading to antimicrobial and anticancer effects. .
Comparison with Similar Compounds
Chemical Identity :
- IUPAC Name: 7-Hydroxyquinoline-3-carboxylic acid
- CAS No.: 659730-27-5
- Molecular Formula: C₁₀H₇NO₃
- Molecular Weight : 189.17 g/mol
- Structure: A quinoline backbone substituted with a hydroxyl (-OH) group at position 7 and a carboxylic acid (-COOH) group at position 3 .
Synthesis: Produced via hydrolysis of ethyl 7-methoxyquinoline-3-carboxylate using aqueous HBr (48%) at 105°C for 88.5 hours, yielding 90% product .
Physicochemical Properties :
- Appearance : Brown solid.
- Safety Profile : Classified with hazard codes H302 (harmful if swallowed), H315 (skin irritation), H319 (eye irritation), and H335 (respiratory irritation) .
Comparison with Structurally Similar Compounds
Positional Isomers: Hydroxyquinoline-3-carboxylic Acids
Functional Group Variants: Quinoline-3-carboxylic Acid Derivatives
Complex Derivatives with Pharmaceutical Relevance
Key Research Findings
Physicochemical Behavior
- Acidity: The -OH group at C7 in this compound increases acidity compared to methyl or chloro derivatives, influencing solubility and metal-binding capacity .
- Thermal Stability: Chloro and methyl substituents (e.g., in 7-chloro-8-methylquinoline-3-carboxylic acid) enhance thermal stability, whereas hydroxyl groups may reduce it due to hydrogen bonding .
Biological Activity
7-Hydroxyquinoline-3-carboxylic acid (7-HQCA) is a compound belonging to the quinoline family, characterized by its heterocyclic aromatic structure. This compound has garnered attention due to its diverse biological activities and potential applications in medicinal chemistry, organic synthesis, and material science. This article explores the biological activity of 7-HQCA, including its mechanisms of action, therapeutic potential, and relevant case studies.
Chemical Structure and Properties
The molecular formula of 7-HQCA is CHNO, with a molecular weight of approximately 189.17 g/mol. The structure features a hydroxyl group at the 7th position and a carboxylic acid group at the 3rd position of the quinoline ring, which influences its reactivity and biological properties.
Biological Activities
Research indicates that 7-HQCA exhibits several significant biological activities:
- Antimicrobial Properties : Quinoline derivatives, including 7-HQCA, are known for their antibacterial and antifungal properties. Studies have shown that compounds within this class can inhibit various pathogens, suggesting potential use in treating infections .
- Enzyme Inhibition : 7-HQCA has been studied for its ability to interact with biological macromolecules, particularly enzymes. It has demonstrated inhibitory effects on enzymes involved in cellular respiration, such as malate dehydrogenase . This inhibition can disrupt metabolic pathways in cancer cells, indicating its potential as an anticancer agent.
- Anticancer Activity : Preliminary studies suggest that 7-HQCA may influence cellular pathways related to cancer progression. Its ability to inhibit key enzymes involved in metabolism could lead to reduced growth rates of cancer cells .
The biological activity of 7-HQCA can be attributed to several mechanisms:
- Interaction with DNA : Compounds similar to 7-HQCA can form adducts with DNA, leading to replication arrest and apoptosis in cancer cells .
- Radical Scavenging : The presence of hydroxyl groups allows for radical scavenging activity, which may protect cells from oxidative stress .
- Modulation of Signaling Pathways : Research indicates that 7-HQCA may modulate signaling pathways involved in cell survival and proliferation, further implicating its role in cancer therapy.
Case Studies
Several studies have evaluated the biological activity of 7-HQCA:
- Study on Antimicrobial Effects : One study reported that derivatives of quinoline exhibited significant antimicrobial activity against Pseudomonas aeruginosa and Klebsiella pneumonia, with inhibition zones comparable to standard antibiotics . This underscores the potential application of 7-HQCA in developing new antimicrobial agents.
- Inhibition of Cellular Respiration : Research conducted on various substituted quinoline derivatives demonstrated a direct correlation between structural modifications and their ability to inhibit cellular respiration in Ehrlich ascites cells. This study highlighted the importance of functional groups in enhancing biological activity .
Comparative Analysis with Related Compounds
The following table summarizes the structural similarities and unique features of compounds related to 7-HQCA:
Compound Name | Similarity | Unique Features |
---|---|---|
6-Hydroxyquinoline-3-carboxylic acid | 0.99 | Hydroxyl group at the 6th position |
Ethyl 6-hydroxyquinoline-3-carboxylate | 0.93 | Ethyl ester functionality |
4-Hydroxyquinoline-3-carboxylic acid | 0.90 | Hydroxyl group at the 4th position |
4-Hydroxy-6-methoxyquinoline-3-carboxylic acid | 0.89 | Methoxy group at the 6th position |
8-Hydroxyquinoline-6-carboxylic acid | 0.85 | Hydroxyl group at the 8th position |
This table illustrates that while these compounds share structural characteristics, their differing functional groups provide distinct chemical properties and biological activities.
Q & A
Q. Basic: What are the optimal synthetic routes for 7-Hydroxyquinoline-3-carboxylic acid?
Methodological Answer:
The synthesis typically involves multi-step reactions starting with nitro-substituted quinoline precursors. A validated approach includes:
Nitro Group Reduction : Reduce 8-nitro-1,4-dihydroquinoline-3-carboxylic acid derivatives using catalytic hydrogenation or chemical reductants (e.g., Fe/HCl) to yield 8-amino intermediates .
Cyclization : Perform acid-catalyzed lactamization (e.g., using polyphosphoric acid, PPA) to form the hydroxyquinoline core .
Functional Group Modification : Introduce hydroxyl groups at position 7 via hydrolysis or oxidation of halogenated intermediates (e.g., 7-chloro derivatives) under controlled pH and temperature .
Key Considerations : Monitor reaction progress via TLC or HPLC. Purify intermediates via recrystallization or column chromatography to minimize byproducts.
Q. Advanced: How can researchers resolve contradictions in spectral data when characterizing substituent effects on this compound derivatives?
Methodological Answer:
Contradictions often arise from tautomerism, solvent effects, or protonation states. To resolve these:
Multi-Technique Analysis : Combine -NMR, -NMR, and IR spectroscopy to cross-verify functional groups. For example, IR carbonyl stretches (1680–1720 cm) confirm carboxylic acid tautomers .
DFT Calculations : Use density-functional theory (DFT) with hybrid functionals (e.g., B3LYP) incorporating exact exchange terms to model electronic structures and predict NMR/IR spectra. Compare computed vs. experimental data to identify dominant tautomers .
Solvent Studies : Analyze spectral shifts in polar (DMSO) vs. nonpolar (CDCl) solvents to assess hydrogen bonding and tautomeric equilibria .
Q. Basic: What are the recommended handling and storage protocols for this compound?
Methodological Answer:
Handling :
- Use chemical-resistant gloves (e.g., nitrile) and safety goggles to prevent skin/eye contact .
- Work in a fume hood to avoid inhalation of dust or vapors .
Storage :
- Keep containers tightly sealed in a dry, well-ventilated area at 2–8°C .
- Avoid exposure to moisture and oxidizing agents to prevent degradation .
Q. Advanced: Which computational methods are most effective for studying the electronic properties of this compound?
Methodological Answer:
DFT with Hybrid Functionals : Employ B3LYP or M06-2X functionals with a 6-311++G(d,p) basis set to model ground-state geometries and frontier molecular orbitals (HOMO/LUMO). These methods account for exact exchange and correlation effects critical for quinoline systems .
Solvent Modeling : Use the polarizable continuum model (PCM) to simulate solvent effects on electronic transitions and acidity (pK) .
Reactivity Predictions : Calculate Fukui indices to identify nucleophilic/electrophilic sites for functionalization .
Q. Basic: How should researchers select solvents for solubility testing of this compound derivatives?
Methodological Answer:
Polarity Gradients : Test solubility in solvents with increasing polarity (e.g., hexane → ethyl acetate → DMSO). The carboxylic acid group enhances solubility in polar aprotic solvents (e.g., DMF, DMSO) .
pH-Dependent Solubility : Adjust pH using NaOH (to deprotonate the carboxylic acid) or HCl (to protonate the quinoline nitrogen) .
Co-Solvent Systems : For poorly soluble derivatives, use water-miscible co-solvents (e.g., ethanol-water mixtures) .
Q. Advanced: What strategies are used to analyze structure-activity relationships (SAR) for the antibacterial activity of this compound analogs?
Methodological Answer:
Substituent Variation : Synthesize derivatives with halogen (Cl, F), alkyl (ethyl), or aryl groups at positions 6, 7, or 2. Assess minimum inhibitory concentrations (MICs) against Gram-negative/-positive bacteria .
Molecular Docking : Use AutoDock Vina to model interactions with bacterial DNA gyrase or topoisomerase IV. Focus on hydrogen bonding with key residues (e.g., Ser-83 in E. coli gyrase) .
Metabolic Stability : Perform in vitro microsomal assays (e.g., human liver microsomes) to evaluate cytochrome P450-mediated degradation .
Q. Basic: What analytical techniques are essential for purity assessment of this compound?
Methodological Answer:
HPLC : Use a C18 column with UV detection (254 nm) and a mobile phase of acetonitrile/0.1% formic acid. Purity >98% is typical for research-grade material .
Melting Point : Determine melting range (e.g., 210–215°C) to detect polymorphic impurities .
Elemental Analysis : Confirm C, H, N content within ±0.4% of theoretical values .
Q. Advanced: How can researchers address conflicting bioactivity data in different bacterial strains for this compound derivatives?
Methodological Answer:
Strain-Specific Resistance : Test efflux pump inhibitors (e.g., phenylalanine-arginine β-naphthylamide) to determine if resistance mechanisms (e.g., AcrAB-TolC in E. coli) reduce activity .
Membrane Permeability : Measure compound uptake using fluorescent probes (e.g., ethidium bromide displacement) in outer membrane-deficient mutants .
Gene Knockout Studies : Use CRISPR-Cas9 to delete target genes (e.g., gyrA) and confirm mode of action .
Properties
IUPAC Name |
7-hydroxyquinoline-3-carboxylic acid | |
---|---|---|
Source | PubChem | |
URL | https://pubchem.ncbi.nlm.nih.gov | |
Description | Data deposited in or computed by PubChem | |
InChI |
InChI=1S/C10H7NO3/c12-8-2-1-6-3-7(10(13)14)5-11-9(6)4-8/h1-5,12H,(H,13,14) | |
Source | PubChem | |
URL | https://pubchem.ncbi.nlm.nih.gov | |
Description | Data deposited in or computed by PubChem | |
InChI Key |
RFVSBXGQVAGYJQ-UHFFFAOYSA-N | |
Source | PubChem | |
URL | https://pubchem.ncbi.nlm.nih.gov | |
Description | Data deposited in or computed by PubChem | |
Canonical SMILES |
C1=CC(=CC2=NC=C(C=C21)C(=O)O)O | |
Source | PubChem | |
URL | https://pubchem.ncbi.nlm.nih.gov | |
Description | Data deposited in or computed by PubChem | |
Molecular Formula |
C10H7NO3 | |
Source | PubChem | |
URL | https://pubchem.ncbi.nlm.nih.gov | |
Description | Data deposited in or computed by PubChem | |
DSSTOX Substance ID |
DTXSID00631111 | |
Record name | 7-Oxo-1,7-dihydroquinoline-3-carboxylic acid | |
Source | EPA DSSTox | |
URL | https://comptox.epa.gov/dashboard/DTXSID00631111 | |
Description | DSSTox provides a high quality public chemistry resource for supporting improved predictive toxicology. | |
Molecular Weight |
189.17 g/mol | |
Source | PubChem | |
URL | https://pubchem.ncbi.nlm.nih.gov | |
Description | Data deposited in or computed by PubChem | |
CAS No. |
659730-27-5 | |
Record name | 7-Oxo-1,7-dihydroquinoline-3-carboxylic acid | |
Source | EPA DSSTox | |
URL | https://comptox.epa.gov/dashboard/DTXSID00631111 | |
Description | DSSTox provides a high quality public chemistry resource for supporting improved predictive toxicology. | |
Record name | 7-hydroxyquinoline-3-carboxylic acid | |
Source | European Chemicals Agency (ECHA) | |
URL | https://echa.europa.eu/information-on-chemicals | |
Description | The European Chemicals Agency (ECHA) is an agency of the European Union which is the driving force among regulatory authorities in implementing the EU's groundbreaking chemicals legislation for the benefit of human health and the environment as well as for innovation and competitiveness. | |
Explanation | Use of the information, documents and data from the ECHA website is subject to the terms and conditions of this Legal Notice, and subject to other binding limitations provided for under applicable law, the information, documents and data made available on the ECHA website may be reproduced, distributed and/or used, totally or in part, for non-commercial purposes provided that ECHA is acknowledged as the source: "Source: European Chemicals Agency, http://echa.europa.eu/". Such acknowledgement must be included in each copy of the material. ECHA permits and encourages organisations and individuals to create links to the ECHA website under the following cumulative conditions: Links can only be made to webpages that provide a link to the Legal Notice page. | |
Synthesis routes and methods
Procedure details
Retrosynthesis Analysis
AI-Powered Synthesis Planning: Our tool employs the Template_relevance Pistachio, Template_relevance Bkms_metabolic, Template_relevance Pistachio_ringbreaker, Template_relevance Reaxys, Template_relevance Reaxys_biocatalysis model, leveraging a vast database of chemical reactions to predict feasible synthetic routes.
One-Step Synthesis Focus: Specifically designed for one-step synthesis, it provides concise and direct routes for your target compounds, streamlining the synthesis process.
Accurate Predictions: Utilizing the extensive PISTACHIO, BKMS_METABOLIC, PISTACHIO_RINGBREAKER, REAXYS, REAXYS_BIOCATALYSIS database, our tool offers high-accuracy predictions, reflecting the latest in chemical research and data.
Strategy Settings
Precursor scoring | Relevance Heuristic |
---|---|
Min. plausibility | 0.01 |
Model | Template_relevance |
Template Set | Pistachio/Bkms_metabolic/Pistachio_ringbreaker/Reaxys/Reaxys_biocatalysis |
Top-N result to add to graph | 6 |
Feasible Synthetic Routes
Disclaimer and Information on In-Vitro Research Products
Please be aware that all articles and product information presented on BenchChem are intended solely for informational purposes. The products available for purchase on BenchChem are specifically designed for in-vitro studies, which are conducted outside of living organisms. In-vitro studies, derived from the Latin term "in glass," involve experiments performed in controlled laboratory settings using cells or tissues. It is important to note that these products are not categorized as medicines or drugs, and they have not received approval from the FDA for the prevention, treatment, or cure of any medical condition, ailment, or disease. We must emphasize that any form of bodily introduction of these products into humans or animals is strictly prohibited by law. It is essential to adhere to these guidelines to ensure compliance with legal and ethical standards in research and experimentation.