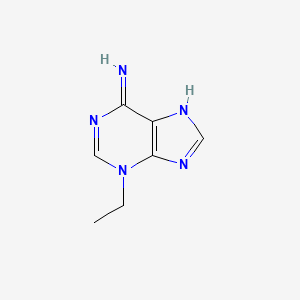
3-Ethyladenine
Overview
Description
3-Ethyladenine is a derivative of adenine, a purine nucleobase that plays a crucial role in the structure of DNA and RNA. The compound is characterized by the substitution of an ethyl group at the third position of the adenine ring. This modification can significantly alter the chemical and biological properties of the molecule, making it a subject of interest in various fields of scientific research.
Preparation Methods
Synthetic Routes and Reaction Conditions: The synthesis of 3-Ethyladenine typically involves the alkylation of adenine. One common method is the reaction of adenine with ethyl iodide in the presence of a base such as potassium carbonate. The reaction is usually carried out in an aprotic solvent like dimethylformamide (DMF) at elevated temperatures to facilitate the alkylation process.
Industrial Production Methods: While specific industrial production methods for this compound are not well-documented, the general approach would involve scaling up the laboratory synthesis methods. This would include optimizing reaction conditions to maximize yield and purity, as well as implementing purification techniques such as recrystallization or chromatography.
Chemical Reactions Analysis
Types of Reactions: 3-Ethyladenine can undergo various chemical reactions, including:
Oxidation: The ethyl group can be oxidized to form aldehydes or carboxylic acids.
Reduction: Reduction reactions can target the adenine ring or the ethyl group, depending on the reagents used.
Substitution: The ethyl group can be substituted with other functional groups through nucleophilic substitution reactions.
Common Reagents and Conditions:
Oxidation: Common oxidizing agents include potassium permanganate (KMnO₄) and chromium trioxide (CrO₃).
Reduction: Reducing agents such as lithium aluminum hydride (LiAlH₄) or sodium borohydride (NaBH₄) are typically used.
Substitution: Nucleophiles like halides or amines can be used in substitution reactions, often in the presence of a catalyst or under basic conditions.
Major Products: The major products of these reactions depend on the specific conditions and reagents used. For example, oxidation of the ethyl group can yield ethyl aldehyde or ethanoic acid, while substitution reactions can introduce various functional groups to the adenine ring.
Scientific Research Applications
3-Ethyladenine has several applications in scientific research:
Chemistry: It is used as a model compound to study the effects of alkylation on nucleobases and their interactions with other molecules.
Biology: Researchers use this compound to investigate DNA repair mechanisms, as alkylated bases can cause mutations and are subject to repair by cellular enzymes.
Medicine: The compound is studied for its potential role in cancer research, particularly in understanding how alkylating agents can induce mutations and affect cellular processes.
Industry: While not widely used in industrial applications, this compound can serve as a precursor for the synthesis of other biologically active compounds.
Mechanism of Action
The mechanism by which 3-Ethyladenine exerts its effects is primarily through its interaction with DNA. The ethyl group can cause structural distortions in the DNA helix, leading to mutations during replication. These mutations can activate DNA repair pathways, providing insights into the cellular mechanisms that maintain genomic integrity. The compound can also interact with various enzymes involved in DNA synthesis and repair, affecting their activity and function.
Comparison with Similar Compounds
3-Methyladenine: Similar to 3-Ethyladenine but with a methyl group instead of an ethyl group. It is widely used as an autophagy inhibitor in biological research.
3-Propyladenine: Contains a propyl group at the third position, offering a longer alkyl chain compared to this compound.
6-Ethyladenine: The ethyl group is attached at the sixth position of the adenine ring, leading to different chemical and biological properties.
Uniqueness: this compound is unique due to the specific position and size of its ethyl group, which can influence its reactivity and interactions with biological molecules. This makes it a valuable tool for studying the effects of alkylation on nucleobases and their role in various cellular processes.
Properties
IUPAC Name |
3-ethyl-7H-purin-6-imine | |
---|---|---|
Source | PubChem | |
URL | https://pubchem.ncbi.nlm.nih.gov | |
Description | Data deposited in or computed by PubChem | |
InChI |
InChI=1S/C7H9N5/c1-2-12-4-11-6(8)5-7(12)10-3-9-5/h3-4,8H,2H2,1H3,(H,9,10) | |
Source | PubChem | |
URL | https://pubchem.ncbi.nlm.nih.gov | |
Description | Data deposited in or computed by PubChem | |
InChI Key |
XAZTXNQITCBSCJ-UHFFFAOYSA-N | |
Source | PubChem | |
URL | https://pubchem.ncbi.nlm.nih.gov | |
Description | Data deposited in or computed by PubChem | |
Canonical SMILES |
CCN1C=NC(=N)C2=C1N=CN2 | |
Source | PubChem | |
URL | https://pubchem.ncbi.nlm.nih.gov | |
Description | Data deposited in or computed by PubChem | |
Molecular Formula |
C7H9N5 | |
Source | PubChem | |
URL | https://pubchem.ncbi.nlm.nih.gov | |
Description | Data deposited in or computed by PubChem | |
DSSTOX Substance ID |
DTXSID10962847 | |
Record name | 3-Ethyl-3H-purin-6-amine | |
Source | EPA DSSTox | |
URL | https://comptox.epa.gov/dashboard/DTXSID10962847 | |
Description | DSSTox provides a high quality public chemistry resource for supporting improved predictive toxicology. | |
Molecular Weight |
163.18 g/mol | |
Source | PubChem | |
URL | https://pubchem.ncbi.nlm.nih.gov | |
Description | Data deposited in or computed by PubChem | |
CAS No. |
43003-87-8 | |
Record name | 3-Ethyladenine | |
Source | ChemIDplus | |
URL | https://pubchem.ncbi.nlm.nih.gov/substance/?source=chemidplus&sourceid=0043003878 | |
Description | ChemIDplus is a free, web search system that provides access to the structure and nomenclature authority files used for the identification of chemical substances cited in National Library of Medicine (NLM) databases, including the TOXNET system. | |
Record name | 3-Ethyl-3H-purin-6-amine | |
Source | EPA DSSTox | |
URL | https://comptox.epa.gov/dashboard/DTXSID10962847 | |
Description | DSSTox provides a high quality public chemistry resource for supporting improved predictive toxicology. | |
Retrosynthesis Analysis
AI-Powered Synthesis Planning: Our tool employs the Template_relevance Pistachio, Template_relevance Bkms_metabolic, Template_relevance Pistachio_ringbreaker, Template_relevance Reaxys, Template_relevance Reaxys_biocatalysis model, leveraging a vast database of chemical reactions to predict feasible synthetic routes.
One-Step Synthesis Focus: Specifically designed for one-step synthesis, it provides concise and direct routes for your target compounds, streamlining the synthesis process.
Accurate Predictions: Utilizing the extensive PISTACHIO, BKMS_METABOLIC, PISTACHIO_RINGBREAKER, REAXYS, REAXYS_BIOCATALYSIS database, our tool offers high-accuracy predictions, reflecting the latest in chemical research and data.
Strategy Settings
Precursor scoring | Relevance Heuristic |
---|---|
Min. plausibility | 0.01 |
Model | Template_relevance |
Template Set | Pistachio/Bkms_metabolic/Pistachio_ringbreaker/Reaxys/Reaxys_biocatalysis |
Top-N result to add to graph | 6 |
Feasible Synthetic Routes
Disclaimer and Information on In-Vitro Research Products
Please be aware that all articles and product information presented on BenchChem are intended solely for informational purposes. The products available for purchase on BenchChem are specifically designed for in-vitro studies, which are conducted outside of living organisms. In-vitro studies, derived from the Latin term "in glass," involve experiments performed in controlled laboratory settings using cells or tissues. It is important to note that these products are not categorized as medicines or drugs, and they have not received approval from the FDA for the prevention, treatment, or cure of any medical condition, ailment, or disease. We must emphasize that any form of bodily introduction of these products into humans or animals is strictly prohibited by law. It is essential to adhere to these guidelines to ensure compliance with legal and ethical standards in research and experimentation.