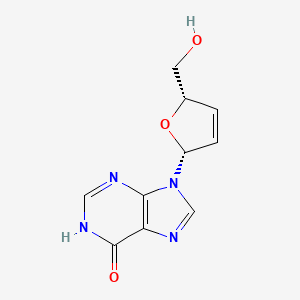
2',3'-Dideoxy-2',3'-didehydroinosine
Overview
Description
2’,3’-Dideoxy-2’,3’-didehydroinosine is a synthetic nucleoside analog that has garnered significant attention due to its potent antiviral properties, particularly against HIV. This compound is structurally similar to inosine but lacks the hydroxyl groups at the 2’ and 3’ positions on the ribose ring, which are replaced by hydrogen atoms and a double bond, respectively .
Biochemical Analysis
Biochemical Properties
2’,3’-Dideoxy-2’,3’-didehydroinosine plays a significant role in biochemical reactions, particularly in the inhibition of viral replication. It interacts with various enzymes and proteins involved in nucleic acid metabolism. One of the primary enzymes it interacts with is reverse transcriptase, an enzyme crucial for the replication of retroviruses. By incorporating into the viral DNA during replication, 2’,3’-Dideoxy-2’,3’-didehydroinosine acts as a chain terminator, preventing the elongation of the DNA strand and thereby inhibiting viral replication . Additionally, it may interact with other nucleoside kinases and phosphatases, influencing their activity and altering nucleotide pools within the cell .
Cellular Effects
The effects of 2’,3’-Dideoxy-2’,3’-didehydroinosine on various cell types and cellular processes are profound. In infected cells, it disrupts the normal function of reverse transcriptase, leading to the inhibition of viral replication. This compound also affects cell signaling pathways and gene expression by interfering with the synthesis of viral DNA. Furthermore, it can influence cellular metabolism by altering the balance of nucleotides within the cell, potentially leading to mitochondrial dysfunction and other metabolic disturbances .
Molecular Mechanism
At the molecular level, 2’,3’-Dideoxy-2’,3’-didehydroinosine exerts its effects primarily through the inhibition of reverse transcriptase. The compound is phosphorylated by cellular kinases to its active triphosphate form, which competes with natural nucleotides for incorporation into the viral DNA. Once incorporated, it acts as a chain terminator due to the absence of the 3’ hydroxyl group, which is necessary for the formation of phosphodiester bonds between nucleotides. This results in the premature termination of DNA synthesis, effectively halting viral replication . Additionally, 2’,3’-Dideoxy-2’,3’-didehydroinosine may inhibit other enzymes involved in nucleotide metabolism, further contributing to its antiviral effects .
Temporal Effects in Laboratory Settings
In laboratory settings, the effects of 2’,3’-Dideoxy-2’,3’-didehydroinosine have been observed to change over time. The compound is relatively stable under standard storage conditions, but its activity can degrade over extended periods or under harsh conditions. Long-term studies have shown that prolonged exposure to 2’,3’-Dideoxy-2’,3’-didehydroinosine can lead to cumulative effects on cellular function, including mitochondrial toxicity and altered gene expression . These temporal effects are crucial for understanding the long-term implications of its use in therapeutic settings.
Dosage Effects in Animal Models
The effects of 2’,3’-Dideoxy-2’,3’-didehydroinosine vary with different dosages in animal models. At therapeutic doses, it effectively inhibits viral replication with minimal toxicity. At higher doses, it can cause significant adverse effects, including hepatotoxicity and mitochondrial dysfunction . Threshold effects have been observed, where doses above a certain level lead to a rapid increase in toxicity, highlighting the importance of careful dosage management in clinical applications.
Metabolic Pathways
2’,3’-Dideoxy-2’,3’-didehydroinosine is involved in several metabolic pathways, primarily those related to nucleotide metabolism. It is phosphorylated by cellular kinases to its active triphosphate form, which then competes with natural nucleotides for incorporation into DNA. This competition can alter metabolic flux and affect the levels of various metabolites within the cell . Additionally, the compound may interact with enzymes such as nucleoside diphosphate kinase and phosphatases, influencing their activity and further modulating nucleotide pools .
Transport and Distribution
Within cells and tissues, 2’,3’-Dideoxy-2’,3’-didehydroinosine is transported and distributed through various mechanisms. It can be taken up by nucleoside transporters, which facilitate its entry into cells. Once inside, it is distributed to different cellular compartments, including the nucleus and mitochondria, where it exerts its effects . The compound’s distribution is influenced by its interactions with binding proteins and its affinity for specific cellular structures.
Preparation Methods
Synthetic Routes and Reaction Conditions: The synthesis of 2’,3’-Dideoxy-2’,3’-didehydroinosine typically involves the radical deoxygenation of ribonucleosides. One common method includes the use of xanthate derivatives, which undergo radical deoxygenation using tris(trimethylsilyl)silane and 1,1’-azobis(cyclohexanecarbonitrile) as reagents . The process also involves the use of bromoethane or 3-bromopropanenitrile as alkylating agents to prepare the ribonucleoside 2’,3’-bisxanthates .
Industrial Production Methods: Industrial production of 2’,3’-Dideoxy-2’,3’-didehydroinosine follows similar synthetic routes but on a larger scale. The use of environmentally friendly and low-cost reagents is emphasized to ensure sustainability and cost-effectiveness .
Chemical Reactions Analysis
Types of Reactions: 2’,3’-Dideoxy-2’,3’-didehydroinosine undergoes various chemical reactions, including:
Oxidation: The compound can be oxidized to form different derivatives.
Reduction: Reduction reactions can convert it back to its parent nucleoside.
Substitution: It can undergo nucleophilic substitution reactions, particularly at the 2’ and 3’ positions.
Common Reagents and Conditions:
Oxidation: Common oxidizing agents include potassium permanganate and chromium trioxide.
Reduction: Reducing agents such as sodium borohydride and lithium aluminum hydride are used.
Substitution: Nucleophiles like ammonia and amines are commonly employed.
Major Products: The major products formed from these reactions include various derivatives of 2’,3’-Dideoxy-2’,3’-didehydroinosine, which can have different biological activities .
Scientific Research Applications
2’,3’-Dideoxy-2’,3’-didehydroinosine has a wide range of applications in scientific research:
Chemistry: It is used as a building block for the synthesis of other nucleoside analogs.
Biology: The compound is studied for its effects on cellular processes and its potential as a tool for genetic research.
Medicine: It is primarily known for its antiviral properties, particularly against HIV.
Industry: The compound is used in the development of antiviral drugs and other therapeutic agents.
Mechanism of Action
2’,3’-Dideoxy-2’,3’-didehydroinosine exerts its antiviral effects by inhibiting the reverse transcriptase enzyme of HIV. Once inside the cell, it is phosphorylated to its active triphosphate form, which competes with natural nucleotides for incorporation into the viral DNA. This incorporation results in chain termination, effectively halting viral replication .
Comparison with Similar Compounds
2’,3’-Dideoxyadenosine: Another nucleoside analog with similar antiviral properties.
2’,3’-Dideoxycytidine: Known for its use in HIV treatment.
2’,3’-Didehydro-2’,3’-dideoxythymidine: Also used as an antiviral agent.
Uniqueness: 2’,3’-Dideoxy-2’,3’-didehydroinosine is unique due to its specific structure, which allows it to effectively inhibit HIV reverse transcriptase. Its lack of hydroxyl groups at the 2’ and 3’ positions and the presence of a double bond confer distinct chemical properties that enhance its antiviral activity .
Properties
IUPAC Name |
9-[(2R,5S)-5-(hydroxymethyl)-2,5-dihydrofuran-2-yl]-1H-purin-6-one | |
---|---|---|
Source | PubChem | |
URL | https://pubchem.ncbi.nlm.nih.gov | |
Description | Data deposited in or computed by PubChem | |
InChI |
InChI=1S/C10H10N4O3/c15-3-6-1-2-7(17-6)14-5-13-8-9(14)11-4-12-10(8)16/h1-2,4-7,15H,3H2,(H,11,12,16)/t6-,7+/m0/s1 | |
Source | PubChem | |
URL | https://pubchem.ncbi.nlm.nih.gov | |
Description | Data deposited in or computed by PubChem | |
InChI Key |
TYQPBHYPIRLWKU-NKWVEPMBSA-N | |
Source | PubChem | |
URL | https://pubchem.ncbi.nlm.nih.gov | |
Description | Data deposited in or computed by PubChem | |
Canonical SMILES |
C1=CC(OC1CO)N2C=NC3=C2N=CNC3=O | |
Source | PubChem | |
URL | https://pubchem.ncbi.nlm.nih.gov | |
Description | Data deposited in or computed by PubChem | |
Isomeric SMILES |
C1=C[C@@H](O[C@@H]1CO)N2C=NC3=C2N=CNC3=O | |
Source | PubChem | |
URL | https://pubchem.ncbi.nlm.nih.gov | |
Description | Data deposited in or computed by PubChem | |
Molecular Formula |
C10H10N4O3 | |
Source | PubChem | |
URL | https://pubchem.ncbi.nlm.nih.gov | |
Description | Data deposited in or computed by PubChem | |
DSSTOX Substance ID |
DTXSID80195544 | |
Record name | 2',3'-Dideoxy-2',3'-didehydroinosine | |
Source | EPA DSSTox | |
URL | https://comptox.epa.gov/dashboard/DTXSID80195544 | |
Description | DSSTox provides a high quality public chemistry resource for supporting improved predictive toxicology. | |
Molecular Weight |
234.21 g/mol | |
Source | PubChem | |
URL | https://pubchem.ncbi.nlm.nih.gov | |
Description | Data deposited in or computed by PubChem | |
CAS No. |
42867-68-5 | |
Record name | 2',3'-Dideoxy-2',3'-didehydroinosine | |
Source | ChemIDplus | |
URL | https://pubchem.ncbi.nlm.nih.gov/substance/?source=chemidplus&sourceid=0042867685 | |
Description | ChemIDplus is a free, web search system that provides access to the structure and nomenclature authority files used for the identification of chemical substances cited in National Library of Medicine (NLM) databases, including the TOXNET system. | |
Record name | 2',3'-Dideoxy-2',3'-didehydroinosine | |
Source | EPA DSSTox | |
URL | https://comptox.epa.gov/dashboard/DTXSID80195544 | |
Description | DSSTox provides a high quality public chemistry resource for supporting improved predictive toxicology. | |
Record name | 2',3'-DIDEOXY-2',3'-DIDEHYDROINOSINE | |
Source | FDA Global Substance Registration System (GSRS) | |
URL | https://gsrs.ncats.nih.gov/ginas/app/beta/substances/849Y7EQ734 | |
Description | The FDA Global Substance Registration System (GSRS) enables the efficient and accurate exchange of information on what substances are in regulated products. Instead of relying on names, which vary across regulatory domains, countries, and regions, the GSRS knowledge base makes it possible for substances to be defined by standardized, scientific descriptions. | |
Explanation | Unless otherwise noted, the contents of the FDA website (www.fda.gov), both text and graphics, are not copyrighted. They are in the public domain and may be republished, reprinted and otherwise used freely by anyone without the need to obtain permission from FDA. Credit to the U.S. Food and Drug Administration as the source is appreciated but not required. | |
Retrosynthesis Analysis
AI-Powered Synthesis Planning: Our tool employs the Template_relevance Pistachio, Template_relevance Bkms_metabolic, Template_relevance Pistachio_ringbreaker, Template_relevance Reaxys, Template_relevance Reaxys_biocatalysis model, leveraging a vast database of chemical reactions to predict feasible synthetic routes.
One-Step Synthesis Focus: Specifically designed for one-step synthesis, it provides concise and direct routes for your target compounds, streamlining the synthesis process.
Accurate Predictions: Utilizing the extensive PISTACHIO, BKMS_METABOLIC, PISTACHIO_RINGBREAKER, REAXYS, REAXYS_BIOCATALYSIS database, our tool offers high-accuracy predictions, reflecting the latest in chemical research and data.
Strategy Settings
Precursor scoring | Relevance Heuristic |
---|---|
Min. plausibility | 0.01 |
Model | Template_relevance |
Template Set | Pistachio/Bkms_metabolic/Pistachio_ringbreaker/Reaxys/Reaxys_biocatalysis |
Top-N result to add to graph | 6 |
Feasible Synthetic Routes
Disclaimer and Information on In-Vitro Research Products
Please be aware that all articles and product information presented on BenchChem are intended solely for informational purposes. The products available for purchase on BenchChem are specifically designed for in-vitro studies, which are conducted outside of living organisms. In-vitro studies, derived from the Latin term "in glass," involve experiments performed in controlled laboratory settings using cells or tissues. It is important to note that these products are not categorized as medicines or drugs, and they have not received approval from the FDA for the prevention, treatment, or cure of any medical condition, ailment, or disease. We must emphasize that any form of bodily introduction of these products into humans or animals is strictly prohibited by law. It is essential to adhere to these guidelines to ensure compliance with legal and ethical standards in research and experimentation.