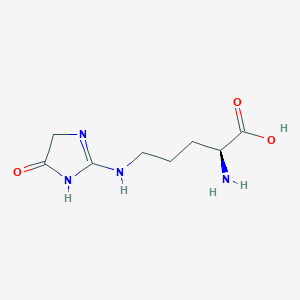
Glyoxal-hydroimidazolone isomer
Overview
Description
Glyoxal-hydroimidazolone isomer is a compound derived from the reaction between glyoxal and amino acids, particularly arginine. This compound is a type of advanced glycation end-product (AGE), which forms through the Maillard reaction, a chemical reaction between amino acids and reducing sugars. Glyoxal-hydroimidazolone isomers are significant in various biological processes and have been studied for their roles in aging, diabetes, and other metabolic disorders .
Biochemical Analysis
Biochemical Properties
Glyoxal-hydroimidazolone isomer plays a significant role in biochemical reactions, particularly in the formation of AGEs. It interacts with various enzymes, proteins, and other biomolecules. For instance, this compound is known to interact with glyoxalase I and glyoxalase II, enzymes involved in the detoxification of glyoxal. These interactions lead to the formation of stable adducts, which can affect the function of the proteins involved . Additionally, this compound can modify proteins by forming cross-links, which can alter their structure and function .
Cellular Effects
This compound has profound effects on various types of cells and cellular processes. It influences cell function by affecting cell signaling pathways, gene expression, and cellular metabolism. For example, in Caenorhabditis elegans, this compound has been shown to increase food intake by altering tyramine signaling via the GATA transcription factor ELT-3 . This compound can also induce oxidative stress and inflammation, leading to cellular damage and apoptosis . Furthermore, this compound can affect the expression of genes involved in detoxification and stress response .
Molecular Mechanism
The molecular mechanism of this compound involves its interaction with biomolecules, enzyme inhibition or activation, and changes in gene expression. This compound exerts its effects by binding to proteins and forming stable adducts, which can inhibit or activate enzyme activity . For instance, it can inhibit the activity of glyoxalase I, leading to the accumulation of glyoxal and increased formation of AGEs . Additionally, this compound can induce changes in gene expression by activating transcription factors such as ELT-3, which regulates the expression of genes involved in tyramine signaling .
Temporal Effects in Laboratory Settings
In laboratory settings, the effects of this compound change over time. The stability and degradation of this compound can influence its long-term effects on cellular function. This compound is relatively stable under physiological conditions, but it can degrade over time, leading to the formation of other reactive intermediates . Long-term exposure to this compound can result in chronic oxidative stress and inflammation, which can affect cellular function and viability .
Dosage Effects in Animal Models
The effects of this compound vary with different dosages in animal models. At low doses, this compound can induce mild oxidative stress and inflammation, which can be beneficial in some contexts by activating stress response pathways . At high doses, this compound can cause severe oxidative damage, inflammation, and apoptosis . Threshold effects have been observed, where the toxic effects of this compound become apparent only above a certain concentration .
Metabolic Pathways
This compound is involved in several metabolic pathways, including the detoxification of glyoxal by the glyoxalase system. This system comprises two enzymes, glyoxalase I and glyoxalase II, and a catalytic amount of glutathione cofactor . This compound can also affect metabolic flux by modifying key metabolic enzymes, leading to changes in metabolite levels . For example, it can inhibit the activity of glycolytic enzymes, resulting in altered glucose metabolism .
Transport and Distribution
This compound is transported and distributed within cells and tissues through various mechanisms. It can interact with transporters and binding proteins, which facilitate its uptake and distribution . Additionally, this compound can accumulate in specific tissues, such as the lens of the eye, where it can contribute to the formation of cataracts . The localization and accumulation of this compound can influence its biological activity and effects .
Subcellular Localization
The subcellular localization of this compound is critical for its activity and function. This compound can be targeted to specific compartments or organelles through targeting signals or post-translational modifications . For instance, this compound can localize to the nucleus, where it can interact with transcription factors and influence gene expression . Additionally, it can accumulate in the mitochondria, leading to mitochondrial dysfunction and oxidative stress .
Preparation Methods
Synthetic Routes and Reaction Conditions: Glyoxal-hydroimidazolone isomers are typically synthesized through the reaction of glyoxal with arginine residues in proteins. The reaction conditions often involve aqueous solutions at physiological pH and temperature. The formation of these isomers can be accelerated by increasing the concentration of glyoxal or by using catalysts that promote the Maillard reaction .
Industrial Production Methods: In industrial settings, the production of glyoxal-hydroimidazolone isomers can be achieved through controlled glycation processes. These processes involve the precise regulation of glyoxal and protein concentrations, as well as the maintenance of optimal reaction conditions to maximize yield and purity .
Chemical Reactions Analysis
Types of Reactions: Glyoxal-hydroimidazolone isomers undergo various chemical reactions, including:
Oxidation: These isomers can be oxidized to form more stable compounds.
Reduction: Reduction reactions can convert glyoxal-hydroimidazolone isomers back to their precursor forms.
Substitution: Substitution reactions can occur at the amino acid residues, leading to the formation of different glycation end-products.
Common Reagents and Conditions:
Oxidation: Common oxidizing agents include hydrogen peroxide and potassium permanganate.
Reduction: Reducing agents such as sodium borohydride are often used.
Substitution: Various nucleophiles can be used to facilitate substitution reactions.
Major Products Formed: The major products formed from these reactions include other advanced glycation end-products, such as argpyrimidine and pentosidine .
Scientific Research Applications
Glyoxal-hydroimidazolone isomers have a wide range of scientific research applications:
Chemistry: They are used as model compounds to study the Maillard reaction and glycation processes.
Biology: Research on these isomers helps in understanding their role in cellular aging and metabolic diseases.
Medicine: Glyoxal-hydroimidazolone isomers are studied for their potential as biomarkers for diabetes and other metabolic disorders.
Industry: They are used in the food industry to study the effects of glycation on food quality and safety.
Mechanism of Action
The mechanism by which glyoxal-hydroimidazolone isomers exert their effects involves the modification of proteins through glycation. This modification alters the structure and function of proteins, leading to changes in cellular signaling pathways. The molecular targets include arginine residues in proteins, and the pathways involved are those related to oxidative stress and inflammation .
Comparison with Similar Compounds
Methylglyoxal-derived hydroimidazolone (MG-H1): Similar in structure and formation process.
Argpyrimidine: Another advanced glycation end-product formed from the reaction of methylglyoxal with arginine.
Pentosidine: Formed from the reaction of reducing sugars with lysine and arginine residues.
Uniqueness: Glyoxal-hydroimidazolone isomers are unique due to their specific formation from glyoxal and their distinct structural properties. They have unique biological effects, particularly in the context of aging and metabolic diseases, which sets them apart from other glycation end-products .
Properties
IUPAC Name |
(2S)-2-amino-5-[(5-oxo-1,4-dihydroimidazol-2-yl)amino]pentanoic acid | |
---|---|---|
Source | PubChem | |
URL | https://pubchem.ncbi.nlm.nih.gov | |
Description | Data deposited in or computed by PubChem | |
InChI |
InChI=1S/C8H14N4O3/c9-5(7(14)15)2-1-3-10-8-11-4-6(13)12-8/h5H,1-4,9H2,(H,14,15)(H2,10,11,12,13)/t5-/m0/s1 | |
Source | PubChem | |
URL | https://pubchem.ncbi.nlm.nih.gov | |
Description | Data deposited in or computed by PubChem | |
InChI Key |
HNPMYKAKAHPOGD-YFKPBYRVSA-N | |
Source | PubChem | |
URL | https://pubchem.ncbi.nlm.nih.gov | |
Description | Data deposited in or computed by PubChem | |
Canonical SMILES |
C1C(=O)NC(=N1)NCCCC(C(=O)O)N | |
Source | PubChem | |
URL | https://pubchem.ncbi.nlm.nih.gov | |
Description | Data deposited in or computed by PubChem | |
Isomeric SMILES |
C1C(=O)NC(=N1)NCCC[C@@H](C(=O)O)N | |
Source | PubChem | |
URL | https://pubchem.ncbi.nlm.nih.gov | |
Description | Data deposited in or computed by PubChem | |
Molecular Formula |
C8H14N4O3 | |
Source | PubChem | |
URL | https://pubchem.ncbi.nlm.nih.gov | |
Description | Data deposited in or computed by PubChem | |
Molecular Weight |
214.22 g/mol | |
Source | PubChem | |
URL | https://pubchem.ncbi.nlm.nih.gov | |
Description | Data deposited in or computed by PubChem | |
Retrosynthesis Analysis
AI-Powered Synthesis Planning: Our tool employs the Template_relevance Pistachio, Template_relevance Bkms_metabolic, Template_relevance Pistachio_ringbreaker, Template_relevance Reaxys, Template_relevance Reaxys_biocatalysis model, leveraging a vast database of chemical reactions to predict feasible synthetic routes.
One-Step Synthesis Focus: Specifically designed for one-step synthesis, it provides concise and direct routes for your target compounds, streamlining the synthesis process.
Accurate Predictions: Utilizing the extensive PISTACHIO, BKMS_METABOLIC, PISTACHIO_RINGBREAKER, REAXYS, REAXYS_BIOCATALYSIS database, our tool offers high-accuracy predictions, reflecting the latest in chemical research and data.
Strategy Settings
Precursor scoring | Relevance Heuristic |
---|---|
Min. plausibility | 0.01 |
Model | Template_relevance |
Template Set | Pistachio/Bkms_metabolic/Pistachio_ringbreaker/Reaxys/Reaxys_biocatalysis |
Top-N result to add to graph | 6 |
Feasible Synthetic Routes
Q1: What are glyoxal-hydroimidazolone isomers (G-H1/H3), and why are they relevant in UHT milk?
A1: Glyoxal-hydroimidazolone isomers (G-H1/H3) are advanced glycation endproducts (AGEs). AGEs form through a series of reactions known as the Maillard reaction, which involves the interaction of sugars like lactose with proteins. In the context of UHT milk, the heat treatment used for sterilization can accelerate the Maillard reaction, leading to the formation of AGEs like G-H1/H3.
Q2: Can G-H1/H3 serve as markers for Maillard reaction progression in UHT milk?
A: Yes, the research suggests that G-H1/H3, along with other AGEs like N-ε-carboxymethyllysine (CML), N-ε-carboxyethyllysine (CEL), and methylglyoxal-hydroimidazolone isomers (MG-H1/H3), can potentially be used as markers to assess the extent of the Maillard reaction in UHT milk. [] The study demonstrated that the concentrations of these AGEs, including G-H1/H3, increased consistently during storage, indicating their potential as indicators of Maillard reaction progression.
Disclaimer and Information on In-Vitro Research Products
Please be aware that all articles and product information presented on BenchChem are intended solely for informational purposes. The products available for purchase on BenchChem are specifically designed for in-vitro studies, which are conducted outside of living organisms. In-vitro studies, derived from the Latin term "in glass," involve experiments performed in controlled laboratory settings using cells or tissues. It is important to note that these products are not categorized as medicines or drugs, and they have not received approval from the FDA for the prevention, treatment, or cure of any medical condition, ailment, or disease. We must emphasize that any form of bodily introduction of these products into humans or animals is strictly prohibited by law. It is essential to adhere to these guidelines to ensure compliance with legal and ethical standards in research and experimentation.