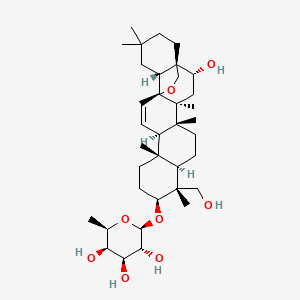
Prosapogenin G
Overview
Description
Prosapogenin G is a triterpenoid saponin, a class of natural products known for their diverse biological activities. These compounds are typically derived from plants and are characterized by their glycosylated triterpene or steroidal core structures. This compound, in particular, has garnered attention for its potential therapeutic applications and its role as a precursor in the synthesis of other bioactive saponins .
Biochemical Analysis
Cellular Effects
Prosapogenin G exerts a range of effects on different cell types and cellular processes. It has been shown to induce apoptosis in cancer cells by inhibiting the STAT3 signaling pathway and glycolysis . This inhibition leads to growth arrest and programmed cell death in cancer cells, highlighting its potential as an anticancer agent. Furthermore, this compound influences cell signaling pathways, gene expression, and cellular metabolism, contributing to its diverse biological activities.
Molecular Mechanism
The molecular mechanism of this compound involves its binding interactions with biomolecules, enzyme inhibition or activation, and changes in gene expression. This compound binds to specific receptors and enzymes, modulating their activity and triggering downstream signaling cascades. For instance, it inhibits the STAT3 signaling pathway, leading to reduced expression of genes involved in cell proliferation and survival . Additionally, this compound affects glycolytic enzymes, thereby altering cellular metabolism and energy production.
Temporal Effects in Laboratory Settings
In laboratory settings, the effects of this compound have been studied over time to understand its stability, degradation, and long-term impact on cellular function. Studies have shown that this compound maintains its bioactivity over extended periods, with minimal degradation under controlled conditions . Long-term exposure to this compound has been associated with sustained inhibition of cancer cell growth and modulation of immune responses, indicating its potential for therapeutic applications.
Dosage Effects in Animal Models
The effects of this compound vary with different dosages in animal models. At lower doses, this compound exhibits beneficial effects such as anti-inflammatory and anticancer activities without significant toxicity . At higher doses, it may induce adverse effects, including hepatotoxicity and gastrointestinal disturbances. These findings underscore the importance of determining optimal dosages for therapeutic use to maximize benefits while minimizing risks.
Metabolic Pathways
This compound is involved in various metabolic pathways, interacting with enzymes and cofactors that regulate its bioactivity. It has been shown to influence the PI3K-Akt signaling pathway, which plays a crucial role in cell survival and metabolism . By modulating this pathway, this compound affects metabolic flux and metabolite levels, contributing to its therapeutic effects.
Transport and Distribution
Within cells and tissues, this compound is transported and distributed through interactions with specific transporters and binding proteins. These interactions facilitate its localization to target sites, where it exerts its biological effects . The compound’s distribution is influenced by factors such as tissue permeability and binding affinity, which determine its accumulation and bioavailability.
Subcellular Localization
This compound exhibits specific subcellular localization, which is essential for its activity and function. It is directed to particular compartments or organelles through targeting signals and post-translational modifications . This localization enables this compound to interact with its molecular targets effectively, thereby modulating cellular processes and exerting its therapeutic effects.
Preparation Methods
Synthetic Routes and Reaction Conditions
Prosapogenin G can be synthesized through enzymatic hydrolysis of ginsenosides, which are major saponins found in the roots of Panax ginseng. A lactase preparation from Penicillium sp. has been shown to hydrolyze ginsenosides Re and Rg1 to produce 20(S)-ginsenoside Rh1, a minor saponin . This enzymatic method is highly efficient and offers a clean alternative to traditional chemical synthesis.
Industrial Production Methods
Industrial production of this compound often involves the extraction and purification of saponin-rich extracts from plants such as Quillaja saponaria. Basic hydrolysis and chromatographic purification are commonly employed to isolate the branched trisaccharide–triterpene conjugate, which is a key intermediate in the production of this compound .
Chemical Reactions Analysis
Types of Reactions
Prosapogenin G undergoes various chemical reactions, including hydrolysis, glycosylation, and oxidation. These reactions are essential for modifying the compound to enhance its biological activity or to produce derivatives with specific properties.
Common Reagents and Conditions
Hydrolysis: Basic hydrolysis using alkaline conditions is commonly used to break down complex saponins into simpler prosapogenins.
Major Products Formed
The major products formed from these reactions include various glycosylated derivatives of this compound, which can exhibit enhanced or altered biological activities compared to the parent compound .
Scientific Research Applications
Mechanism of Action
The mechanism of action of Prosapogenin G involves its interaction with various molecular targets and pathways. One of the key pathways is the inhibition of the STAT3 signaling pathway, which is known to play a crucial role in cancer cell proliferation and survival . By blocking this pathway, this compound induces apoptosis and inhibits the growth of cancer cells. Additionally, the compound modulates glycometabolism-related genes, further contributing to its anticancer effects .
Comparison with Similar Compounds
Prosapogenin G is unique among triterpenoid saponins due to its specific glycosylation pattern and biological activities. Similar compounds include:
Ginsenoside Rh1: Another triterpenoid saponin derived from ginsenosides, known for its anti-inflammatory and anticancer properties.
This compound stands out due to its relatively low toxicity and high efficiency in inducing apoptosis in cancer cells, making it a promising candidate for further therapeutic development .
Properties
IUPAC Name |
(2R,3R,4S,5R,6R)-2-[[(1S,2R,4S,5R,8R,9R,10S,13S,14R,17S,18R)-2-hydroxy-9-(hydroxymethyl)-4,5,9,13,20,20-hexamethyl-24-oxahexacyclo[15.5.2.01,18.04,17.05,14.08,13]tetracos-15-en-10-yl]oxy]-6-methyloxane-3,4,5-triol | |
---|---|---|
Source | PubChem | |
URL | https://pubchem.ncbi.nlm.nih.gov | |
Description | Data deposited in or computed by PubChem | |
InChI |
InChI=1S/C36H58O8/c1-20-26(39)27(40)28(41)29(43-20)44-25-10-11-31(4)21(32(25,5)18-37)8-12-33(6)22(31)9-13-36-23-16-30(2,3)14-15-35(23,19-42-36)24(38)17-34(33,36)7/h9,13,20-29,37-41H,8,10-12,14-19H2,1-7H3/t20-,21-,22-,23-,24-,25+,26+,27+,28-,29+,31+,32+,33-,34+,35-,36+/m1/s1 | |
Source | PubChem | |
URL | https://pubchem.ncbi.nlm.nih.gov | |
Description | Data deposited in or computed by PubChem | |
InChI Key |
WSSVJIGMYVWUJL-PSLKBDIJSA-N | |
Source | PubChem | |
URL | https://pubchem.ncbi.nlm.nih.gov | |
Description | Data deposited in or computed by PubChem | |
Canonical SMILES |
CC1C(C(C(C(O1)OC2CCC3(C(C2(C)CO)CCC4(C3C=CC56C4(CC(C7(C5CC(CC7)(C)C)CO6)O)C)C)C)O)O)O | |
Source | PubChem | |
URL | https://pubchem.ncbi.nlm.nih.gov | |
Description | Data deposited in or computed by PubChem | |
Isomeric SMILES |
C[C@@H]1[C@@H]([C@@H]([C@H]([C@@H](O1)O[C@H]2CC[C@]3([C@H]([C@]2(C)CO)CC[C@@]4([C@@H]3C=C[C@@]56[C@]4(C[C@H]([C@@]7([C@H]5CC(CC7)(C)C)CO6)O)C)C)C)O)O)O | |
Source | PubChem | |
URL | https://pubchem.ncbi.nlm.nih.gov | |
Description | Data deposited in or computed by PubChem | |
Molecular Formula |
C36H58O8 | |
Source | PubChem | |
URL | https://pubchem.ncbi.nlm.nih.gov | |
Description | Data deposited in or computed by PubChem | |
Molecular Weight |
618.8 g/mol | |
Source | PubChem | |
URL | https://pubchem.ncbi.nlm.nih.gov | |
Description | Data deposited in or computed by PubChem | |
Retrosynthesis Analysis
AI-Powered Synthesis Planning: Our tool employs the Template_relevance Pistachio, Template_relevance Bkms_metabolic, Template_relevance Pistachio_ringbreaker, Template_relevance Reaxys, Template_relevance Reaxys_biocatalysis model, leveraging a vast database of chemical reactions to predict feasible synthetic routes.
One-Step Synthesis Focus: Specifically designed for one-step synthesis, it provides concise and direct routes for your target compounds, streamlining the synthesis process.
Accurate Predictions: Utilizing the extensive PISTACHIO, BKMS_METABOLIC, PISTACHIO_RINGBREAKER, REAXYS, REAXYS_BIOCATALYSIS database, our tool offers high-accuracy predictions, reflecting the latest in chemical research and data.
Strategy Settings
Precursor scoring | Relevance Heuristic |
---|---|
Min. plausibility | 0.01 |
Model | Template_relevance |
Template Set | Pistachio/Bkms_metabolic/Pistachio_ringbreaker/Reaxys/Reaxys_biocatalysis |
Top-N result to add to graph | 6 |
Feasible Synthetic Routes
Q1: What are the metabolic pathways of Prosaikogenin G?
A: Prosaikogenin G is a metabolite of Saikosaponin D, formed in the gastrointestinal tract. In rat liver microsomes, Prosaikogenin G undergoes further metabolism primarily through hydroxylation and carboxylation reactions on its aglycone moiety. [] These metabolic reactions lead to the formation of various metabolites. For a detailed metabolic pathway, refer to the study by [Liu et al. (2015)][2].
Q2: How does the structure of Prosaikogenin G relate to its hemolytic activity?
A: Prosaikogenin G, possessing an alpha-hydroxyl function at the C16 position, exhibits strong hemolytic activity. [] This is similar to its precursor, Saikosaponin D. Interestingly, compounds with a beta-hydroxyl function at C16, like Prosaikogenin F and Saikosaponin A, demonstrate weaker hemolytic activity. This suggests that the stereochemistry at the C16 position significantly influences the hemolytic potential of these compounds. [] Additionally, the adsorbability of these compounds onto red blood cell membranes correlates with their hemolytic activity. []
Q3: Can Prosaikogenin G induce corticosterone secretion?
A: While Prosaikogenin G itself shows slight corticosterone secretion-inducing activity, its precursor, Saikosaponin D, and its further metabolite, Saikogenin G, exhibit much stronger activities. [] This highlights the importance of the sugar moiety for this specific biological activity. Interestingly, gastric metabolites of Saikosaponin D and their downstream intestinal metabolites do not demonstrate this effect, emphasizing the specific structural requirements for inducing corticosterone secretion. []
Q4: How can Prosaikogenin G be produced in larger quantities?
A: Prosaikogenin G, along with its precursor Saikogenin G, can be efficiently produced through enzymatic hydrolysis of Saikosaponin D. [] Recombinant β-glycosidases, such as BglPm and BglLk derived from Paenibacillus mucilaginosus and Lactobacillus koreensis respectively, show high activity in cleaving glycosidic bonds in saikosaponins. [] This enzymatic approach offers a promising avenue for large-scale production of Prosaikogenin G and other valuable saikosaponin metabolites for further research and potential applications. []
Disclaimer and Information on In-Vitro Research Products
Please be aware that all articles and product information presented on BenchChem are intended solely for informational purposes. The products available for purchase on BenchChem are specifically designed for in-vitro studies, which are conducted outside of living organisms. In-vitro studies, derived from the Latin term "in glass," involve experiments performed in controlled laboratory settings using cells or tissues. It is important to note that these products are not categorized as medicines or drugs, and they have not received approval from the FDA for the prevention, treatment, or cure of any medical condition, ailment, or disease. We must emphasize that any form of bodily introduction of these products into humans or animals is strictly prohibited by law. It is essential to adhere to these guidelines to ensure compliance with legal and ethical standards in research and experimentation.