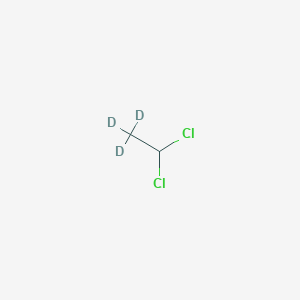
1,1-Dichloroethane (2,2,2-D3)
Overview
Description
1,1-Dichloroethane (2,2,2-D3) is a useful research compound. Its molecular formula is C2H4Cl2 and its molecular weight is 101.97 g/mol. The purity is usually 95%.
BenchChem offers high-quality 1,1-Dichloroethane (2,2,2-D3) suitable for many research applications. Different packaging options are available to accommodate customers' requirements. Please inquire for more information about 1,1-Dichloroethane (2,2,2-D3) including the price, delivery time, and more detailed information at info@benchchem.com.
Mechanism of Action
Target of Action
- 1,1-dichloroethane primarily affects the central nervous system (CNS), liver, and kidneys. In the CNS, it can lead to neurotoxicity, affecting behavior and cognitive function .
Mode of Action
- 1,1-dichloroethane may inhibit aquaporin 4 (AQP4) in astrocytes and myelin basic protein (MBP) in oligodendrocytes, leading to cortical demyelination and neural behavioral changes . These interactions disrupt normal cellular processes, impairing CNS function.
Biochemical Analysis
Biochemical Properties
1,1-Dichloroethane (2,2,2-D3) plays a role in various biochemical reactions, particularly in the context of its interactions with enzymes and proteins. It is known to interact with cytochrome P450 enzymes, which are involved in the metabolism of many xenobiotics. The interaction with these enzymes can lead to the formation of reactive intermediates that may bind covalently to proteins and DNA, potentially causing cellular damage . Additionally, 1,1-Dichloroethane (2,2,2-D3) can induce oxidative stress by generating reactive oxygen species (ROS), which can further interact with cellular biomolecules.
Cellular Effects
The effects of 1,1-Dichloroethane (2,2,2-D3) on various cell types and cellular processes are significant. It has been shown to influence cell signaling pathways, particularly those involved in oxidative stress responses. The compound can alter gene expression by modulating transcription factors such as NF-κB and AP-1, which are critical in the cellular response to stress . Furthermore, 1,1-Dichloroethane (2,2,2-D3) can impact cellular metabolism by inhibiting key metabolic enzymes, leading to altered energy production and metabolic flux.
Molecular Mechanism
At the molecular level, 1,1-Dichloroethane (2,2,2-D3) exerts its effects through several mechanisms. It can bind to and inhibit the activity of cytochrome P450 enzymes, leading to the accumulation of toxic metabolites . Additionally, the compound can form adducts with DNA and proteins, disrupting their normal function. The generation of ROS by 1,1-Dichloroethane (2,2,2-D3) can also lead to oxidative damage to cellular components, further contributing to its toxic effects.
Temporal Effects in Laboratory Settings
In laboratory settings, the effects of 1,1-Dichloroethane (2,2,2-D3) can change over time. The compound is relatively stable under standard storage conditions but can degrade under prolonged exposure to light and heat . Long-term exposure to 1,1-Dichloroethane (2,2,2-D3) in in vitro and in vivo studies has shown that it can cause persistent oxidative stress and chronic cellular damage, leading to potential carcinogenic effects .
Dosage Effects in Animal Models
The effects of 1,1-Dichloroethane (2,2,2-D3) vary with different dosages in animal models. At low doses, the compound may cause mild oxidative stress and transient changes in gene expression. At higher doses, it can lead to significant toxic effects, including liver and kidney damage, central nervous system depression, and increased risk of cancer . Threshold effects have been observed, where the severity of the toxic effects increases sharply beyond a certain dosage level.
Metabolic Pathways
1,1-Dichloroethane (2,2,2-D3) is involved in several metabolic pathways, primarily through its interaction with cytochrome P450 enzymes. These enzymes catalyze the oxidation of 1,1-Dichloroethane (2,2,2-D3), leading to the formation of reactive intermediates that can further react with cellular components . The compound can also affect metabolic flux by inhibiting key metabolic enzymes, leading to altered levels of metabolites and disruption of normal metabolic processes.
Transport and Distribution
Within cells and tissues, 1,1-Dichloroethane (2,2,2-D3) is transported and distributed through passive diffusion and interaction with transport proteins. The compound can accumulate in lipid-rich tissues due to its lipophilic nature, leading to higher local concentrations and potential toxicity . Transporters such as organic anion-transporting polypeptides (OATPs) may also play a role in its cellular uptake and distribution.
Subcellular Localization
The subcellular localization of 1,1-Dichloroethane (2,2,2-D3) can influence its activity and function. The compound is known to localize in the endoplasmic reticulum, where it interacts with cytochrome P450 enzymes . It can also accumulate in mitochondria, leading to mitochondrial dysfunction and impaired energy production. Post-translational modifications and targeting signals may direct 1,1-Dichloroethane (2,2,2-D3) to specific cellular compartments, affecting its overall impact on cellular function.
Biological Activity
1,1-Dichloroethane (2,2,2-D3), also known as deuterated 1,1-dichloroethane, is a chlorinated hydrocarbon that has been the subject of various studies due to its potential biological effects and applications in research. This compound is often used in organic synthesis and as a solvent. Understanding its biological activity is crucial for assessing its safety and potential therapeutic applications.
- Chemical Formula : C₂HCl₂
- Molecular Weight : 98.96 g/mol
- Structure : A colorless, oily liquid with a characteristic sweet odor.
- Solubility : It is moderately soluble in water and highly soluble in organic solvents.
Biological Activity Overview
The biological activity of 1,1-dichloroethane can be categorized into several areas:
- Toxicology : Studies have shown that exposure to 1,1-dichloroethane can lead to various toxic effects. Acute exposure has been associated with respiratory irritation and central nervous system effects. Chronic exposure may result in liver and kidney damage.
- Carcinogenicity : Research indicates that 1,1-dichloroethane may have carcinogenic potential. In animal studies, it has been linked to increased incidences of tumors in the liver and lungs when administered at high doses over prolonged periods .
- Endocrine Disruption : Some studies suggest that dichloroethanes can act as endocrine disruptors. In vitro assays have demonstrated that certain derivatives of dichloroethane exhibit antiestrogenic properties, potentially impacting hormonal balance .
Case Study 1: Toxicological Effects
A study conducted on Sprague-Dawley rats exposed to varying concentrations of 1,1-dichloroethane revealed significant increases in liver tumors at mid to high doses. The study noted a dose-dependent relationship between exposure levels and tumor incidence, highlighting the compound's potential carcinogenicity .
Case Study 2: Endocrine Disruption
In a series of experiments evaluating the antiestrogenic activity of 1,1-dichloro-2,2,3-triarylcyclopropanes (DTACs), it was found that these compounds could inhibit estrogen receptor binding in vitro. One specific compound demonstrated a dose-dependent decrease in uterine weight in immature mice, suggesting significant endocrine-disrupting activity .
Toxicological Studies
Endocrine Activity
Research on the antiestrogenic properties of various dichloroethane derivatives has shown:
Properties
IUPAC Name |
2,2-dichloro-1,1,1-trideuterioethane | |
---|---|---|
Source | PubChem | |
URL | https://pubchem.ncbi.nlm.nih.gov | |
Description | Data deposited in or computed by PubChem | |
InChI |
InChI=1S/C2H4Cl2/c1-2(3)4/h2H,1H3/i1D3 | |
Source | PubChem | |
URL | https://pubchem.ncbi.nlm.nih.gov | |
Description | Data deposited in or computed by PubChem | |
InChI Key |
SCYULBFZEHDVBN-FIBGUPNXSA-N | |
Source | PubChem | |
URL | https://pubchem.ncbi.nlm.nih.gov | |
Description | Data deposited in or computed by PubChem | |
Canonical SMILES |
CC(Cl)Cl | |
Source | PubChem | |
URL | https://pubchem.ncbi.nlm.nih.gov | |
Description | Data deposited in or computed by PubChem | |
Isomeric SMILES |
[2H]C([2H])([2H])C(Cl)Cl | |
Source | PubChem | |
URL | https://pubchem.ncbi.nlm.nih.gov | |
Description | Data deposited in or computed by PubChem | |
Molecular Formula |
C2H4Cl2 | |
Source | PubChem | |
URL | https://pubchem.ncbi.nlm.nih.gov | |
Description | Data deposited in or computed by PubChem | |
Molecular Weight |
101.97 g/mol | |
Source | PubChem | |
URL | https://pubchem.ncbi.nlm.nih.gov | |
Description | Data deposited in or computed by PubChem | |
Retrosynthesis Analysis
AI-Powered Synthesis Planning: Our tool employs the Template_relevance Pistachio, Template_relevance Bkms_metabolic, Template_relevance Pistachio_ringbreaker, Template_relevance Reaxys, Template_relevance Reaxys_biocatalysis model, leveraging a vast database of chemical reactions to predict feasible synthetic routes.
One-Step Synthesis Focus: Specifically designed for one-step synthesis, it provides concise and direct routes for your target compounds, streamlining the synthesis process.
Accurate Predictions: Utilizing the extensive PISTACHIO, BKMS_METABOLIC, PISTACHIO_RINGBREAKER, REAXYS, REAXYS_BIOCATALYSIS database, our tool offers high-accuracy predictions, reflecting the latest in chemical research and data.
Strategy Settings
Precursor scoring | Relevance Heuristic |
---|---|
Min. plausibility | 0.01 |
Model | Template_relevance |
Template Set | Pistachio/Bkms_metabolic/Pistachio_ringbreaker/Reaxys/Reaxys_biocatalysis |
Top-N result to add to graph | 6 |
Feasible Synthetic Routes
Disclaimer and Information on In-Vitro Research Products
Please be aware that all articles and product information presented on BenchChem are intended solely for informational purposes. The products available for purchase on BenchChem are specifically designed for in-vitro studies, which are conducted outside of living organisms. In-vitro studies, derived from the Latin term "in glass," involve experiments performed in controlled laboratory settings using cells or tissues. It is important to note that these products are not categorized as medicines or drugs, and they have not received approval from the FDA for the prevention, treatment, or cure of any medical condition, ailment, or disease. We must emphasize that any form of bodily introduction of these products into humans or animals is strictly prohibited by law. It is essential to adhere to these guidelines to ensure compliance with legal and ethical standards in research and experimentation.