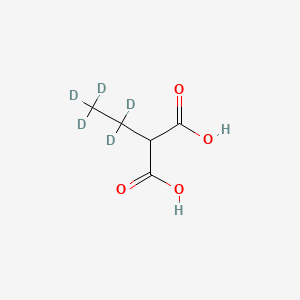
Ethyl-D5-malonic acid
Overview
Description
Ethyl-D5-malonic acid is a deuterated form of ethylmalonic acid, where five hydrogen atoms are replaced with deuterium. This compound is used primarily in research settings to study metabolic pathways and enzyme mechanisms due to its unique isotopic properties .
Mechanism of Action
Target of Action
Ethyl-D5-malonic acid, a derivative of malonic acid, primarily targets the enzyme succinate dehydrogenase (complex II) in the respiratory electron transport chain . This enzyme plays a crucial role in the citric acid cycle, where it facilitates the conversion of succinate into fumarate .
Mode of Action
The interaction of this compound with its target involves a series of reactions. Initially, the compound is deprotonated by a weak base to form an enolate . This enolate then undergoes a nucleophilic substitution reaction with an alkyl halide, forming a new carbon-carbon bond . This process is known as enolate alkylation .
Biochemical Pathways
The action of this compound affects the citric acid cycle, specifically the conversion of succinate to fumarate . The compound acts as a competitive inhibitor of succinate dehydrogenase, binding to the active site of the enzyme without reacting . This prevents the normal substrate, succinate, from binding and being converted into fumarate .
Result of Action
The inhibition of succinate dehydrogenase by this compound disrupts the citric acid cycle, potentially leading to a decrease in the production of ATP . This can have wide-ranging effects at the molecular and cellular levels, impacting energy metabolism within cells .
Biochemical Analysis
Biochemical Properties
Ethyl-D5-malonic acid plays a significant role in biochemical reactions, particularly in the synthesis and degradation of fatty acids. It interacts with several enzymes, including malonyl-CoA decarboxylase and short-chain acyl-CoA dehydrogenase. These interactions are crucial for the regulation of fatty acid metabolism. Malonyl-CoA decarboxylase catalyzes the decarboxylation of malonyl-CoA to acetyl-CoA, while short-chain acyl-CoA dehydrogenase is involved in the beta-oxidation of fatty acids. The presence of this compound can inhibit these enzymes, leading to alterations in metabolic flux and energy production .
Cellular Effects
This compound affects various cellular processes, including cell signaling pathways, gene expression, and cellular metabolism. It has been observed to influence the activity of key metabolic enzymes, leading to changes in the levels of metabolites and energy production. In particular, this compound can disrupt the normal function of mitochondria, leading to altered cellular respiration and ATP production. This disruption can affect cell proliferation, differentiation, and apoptosis .
Molecular Mechanism
The molecular mechanism of this compound involves its interaction with specific enzymes and proteins. It acts as a competitive inhibitor of malonyl-CoA decarboxylase, binding to the active site of the enzyme and preventing the conversion of malonyl-CoA to acetyl-CoA. This inhibition leads to an accumulation of malonyl-CoA, which can further inhibit carnitine palmitoyltransferase I, a key enzyme in fatty acid oxidation. Additionally, this compound can affect gene expression by altering the levels of metabolites that act as signaling molecules .
Temporal Effects in Laboratory Settings
In laboratory settings, the effects of this compound can change over time due to its stability and degradation. The compound is relatively stable under standard storage conditions, but it can degrade over extended periods, leading to a decrease in its efficacy. Long-term studies have shown that this compound can have persistent effects on cellular function, including sustained inhibition of metabolic enzymes and alterations in energy production. These effects can be observed in both in vitro and in vivo studies .
Dosage Effects in Animal Models
The effects of this compound in animal models vary with different dosages. At low doses, the compound can inhibit specific metabolic pathways without causing significant toxicity. At higher doses, this compound can lead to toxic effects, including mitochondrial dysfunction, oxidative stress, and cell death. These adverse effects are dose-dependent and can be observed in various tissues, including the liver, muscle, and brain .
Metabolic Pathways
This compound is involved in several metabolic pathways, including the synthesis and degradation of fatty acids. It interacts with enzymes such as malonyl-CoA decarboxylase and short-chain acyl-CoA dehydrogenase, affecting the levels of key metabolites and the overall metabolic flux. The compound can also influence the levels of intermediates in the tricarboxylic acid cycle, leading to changes in energy production and cellular metabolism .
Transport and Distribution
Within cells and tissues, this compound is transported and distributed through specific transporters and binding proteins. It can be taken up by cells via monocarboxylate transporters and distributed to various cellular compartments. The compound can accumulate in mitochondria, where it exerts its effects on metabolic enzymes and energy production. The distribution of this compound can vary depending on the tissue type and the presence of specific transporters .
Subcellular Localization
This compound is primarily localized in the mitochondria, where it interacts with key metabolic enzymes. The compound can also be found in the cytoplasm and other subcellular compartments, depending on the cell type and metabolic state. The subcellular localization of this compound is influenced by targeting signals and post-translational modifications that direct it to specific organelles. These localization patterns are crucial for its function and effects on cellular metabolism .
Preparation Methods
Synthetic Routes and Reaction Conditions
Ethyl-D5-malonic acid can be synthesized through the malonic ester synthesis. This involves the deprotonation of a di-ester of malonic acid using a weak base, followed by C–C bond formation at the alpha position with an alkyl halide. The ester is then hydrolyzed with aqueous acid, and upon heating, decarboxylation occurs to yield the desired product .
Industrial Production Methods
Large-scale synthesis of this compound involves selective monohydrolysis of symmetric diesters. This method is efficient and environmentally benign, requiring only water, a small proportion of a volatile co-solvent, and inexpensive reagents .
Chemical Reactions Analysis
Types of Reactions
Ethyl-D5-malonic acid undergoes several types of reactions, including:
Acid-Base Reactions: Deprotonation to form enolates.
Nucleophilic Substitution: Enolate nucleophiles react with alkyl halide electrophiles.
Ester Hydrolysis: Conversion of esters to carboxylic acids.
Decarboxylation: Loss of carbon dioxide to form enols
Common Reagents and Conditions
Bases: Sodium ethoxide or methoxide for deprotonation.
Alkyl Halides: Used in nucleophilic substitution reactions.
Aqueous Acid: For ester hydrolysis.
Major Products
The major products formed from these reactions include substituted acetic acids and enols .
Scientific Research Applications
Ethyl-D5-malonic acid is used in various scientific research applications:
Chemistry: Studying reaction mechanisms and isotopic effects.
Biology: Investigating metabolic pathways and enzyme activities.
Medicine: Researching metabolic disorders and potential therapeutic targets.
Industry: Used as a building block in the synthesis of pharmaceuticals and other organic compounds .
Comparison with Similar Compounds
Similar Compounds
Malonic Acid: A dicarboxylic acid with similar reactivity.
Diethyl Malonate: An ester of malonic acid used in similar synthetic procedures.
Acetoacetic Ester: Used in acetoacetic ester synthesis, a process similar to malonic ester synthesis
Uniqueness
Ethyl-D5-malonic acid is unique due to its deuterium content, which makes it valuable for studying isotopic effects and metabolic pathways. Its deuterated form allows for more precise tracking in biochemical studies compared to non-deuterated analogs .
Properties
IUPAC Name |
2-(1,1,2,2,2-pentadeuterioethyl)propanedioic acid | |
---|---|---|
Source | PubChem | |
URL | https://pubchem.ncbi.nlm.nih.gov | |
Description | Data deposited in or computed by PubChem | |
InChI |
InChI=1S/C5H8O4/c1-2-3(4(6)7)5(8)9/h3H,2H2,1H3,(H,6,7)(H,8,9)/i1D3,2D2 | |
Source | PubChem | |
URL | https://pubchem.ncbi.nlm.nih.gov | |
Description | Data deposited in or computed by PubChem | |
InChI Key |
UKFXDFUAPNAMPJ-ZBJDZAJPSA-N | |
Source | PubChem | |
URL | https://pubchem.ncbi.nlm.nih.gov | |
Description | Data deposited in or computed by PubChem | |
Canonical SMILES |
CCC(C(=O)O)C(=O)O | |
Source | PubChem | |
URL | https://pubchem.ncbi.nlm.nih.gov | |
Description | Data deposited in or computed by PubChem | |
Isomeric SMILES |
[2H]C([2H])([2H])C([2H])([2H])C(C(=O)O)C(=O)O | |
Source | PubChem | |
URL | https://pubchem.ncbi.nlm.nih.gov | |
Description | Data deposited in or computed by PubChem | |
Molecular Formula |
C5H8O4 | |
Source | PubChem | |
URL | https://pubchem.ncbi.nlm.nih.gov | |
Description | Data deposited in or computed by PubChem | |
Molecular Weight |
137.15 g/mol | |
Source | PubChem | |
URL | https://pubchem.ncbi.nlm.nih.gov | |
Description | Data deposited in or computed by PubChem | |
Retrosynthesis Analysis
AI-Powered Synthesis Planning: Our tool employs the Template_relevance Pistachio, Template_relevance Bkms_metabolic, Template_relevance Pistachio_ringbreaker, Template_relevance Reaxys, Template_relevance Reaxys_biocatalysis model, leveraging a vast database of chemical reactions to predict feasible synthetic routes.
One-Step Synthesis Focus: Specifically designed for one-step synthesis, it provides concise and direct routes for your target compounds, streamlining the synthesis process.
Accurate Predictions: Utilizing the extensive PISTACHIO, BKMS_METABOLIC, PISTACHIO_RINGBREAKER, REAXYS, REAXYS_BIOCATALYSIS database, our tool offers high-accuracy predictions, reflecting the latest in chemical research and data.
Strategy Settings
Precursor scoring | Relevance Heuristic |
---|---|
Min. plausibility | 0.01 |
Model | Template_relevance |
Template Set | Pistachio/Bkms_metabolic/Pistachio_ringbreaker/Reaxys/Reaxys_biocatalysis |
Top-N result to add to graph | 6 |
Feasible Synthetic Routes
Disclaimer and Information on In-Vitro Research Products
Please be aware that all articles and product information presented on BenchChem are intended solely for informational purposes. The products available for purchase on BenchChem are specifically designed for in-vitro studies, which are conducted outside of living organisms. In-vitro studies, derived from the Latin term "in glass," involve experiments performed in controlled laboratory settings using cells or tissues. It is important to note that these products are not categorized as medicines or drugs, and they have not received approval from the FDA for the prevention, treatment, or cure of any medical condition, ailment, or disease. We must emphasize that any form of bodily introduction of these products into humans or animals is strictly prohibited by law. It is essential to adhere to these guidelines to ensure compliance with legal and ethical standards in research and experimentation.