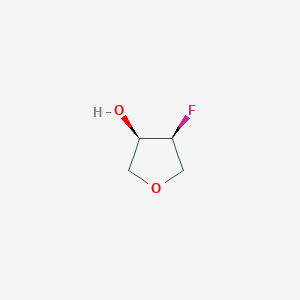
(3R,4S)-4-Fluorotetrahydrofuran-3-ol
Overview
Description
(3R,4S)-4-Fluorotetrahydrofuran-3-ol is a fluorinated tetrahydrofuran derivative characterized by a hydroxyl group at position 3 and a fluorine atom at position 4 in its cyclic ether structure. This stereochemical configuration (3R,4S) imparts distinct electronic and steric properties, making it valuable in pharmaceutical and agrochemical research. The fluorine atom enhances metabolic stability and influences hydrogen-bonding interactions, while the hydroxyl group contributes to solubility and reactivity.
Preparation Methods
Synthetic Routes and Reaction Conditions: (3R,4S)-4-Fluorotetrahydrofuran-3-ol can be synthesized through various methods, including ring-opening reactions of fluorinated epoxides or cyclization of fluorinated diols. These methods typically involve the use of fluorinated precursors and specific catalysts to achieve the desired configuration and purity.
Industrial Production Methods: While detailed industrial production methods are not well-documented, the synthesis of fluorinated tetrahydrofurans generally involves similar principles as laboratory-scale synthesis, with adjustments for scalability, cost-efficiency, and safety. Industrial production may utilize continuous flow reactors and optimized reaction conditions to ensure consistent quality and yield.
Chemical Reactions Analysis
Types of Reactions: (3R,4S)-4-Fluorotetrahydrofuran-3-ol undergoes various chemical reactions, including:
Substitution Reactions: The hydroxyl group (OH) can undergo substitution reactions with suitable reagents, resulting in the formation of new derivatives.
Degradation Reactions: Under certain conditions, it might undergo degradation reactions, such as hydrolysis (cleavage by water) or thermal decomposition at high temperatures.
Common Reagents and Conditions:
Substitution Reactions: Common reagents include halogenating agents, acids, and bases.
Degradation Reactions: Conditions such as acidic or basic environments, elevated temperatures, and the presence of catalysts can facilitate these reactions.
Major Products Formed:
Substitution Reactions: New fluorinated derivatives with different functional groups.
Degradation Reactions: Smaller organic molecules and inorganic by-products, depending on the specific reaction conditions.
Scientific Research Applications
Chemistry: Used as a building block for the synthesis of more complex fluorinated compounds.
Biology: Investigated for its potential interactions with biological molecules and its effects on biological systems.
Medicine: Explored for its potential use in drug development and as a precursor for pharmaceutical compounds.
Industry: Utilized in the development of new materials and as a reagent in various industrial processes.
Mechanism of Action
Currently, there is no documented information regarding the specific mechanism of action of (3R,4S)-4-Fluorotetrahydrofuran-3-ol in biological systems. its unique structure, featuring both a fluorine atom and a hydroxyl group, suggests that it may interact with biological molecules through hydrogen bonding, hydrophobic interactions, and other non-covalent interactions.
Comparison with Similar Compounds
Comparison with Structurally Similar Compounds
The following compounds share core tetrahydrofuran scaffolds but differ in substituents, stereochemistry, and functional groups, leading to divergent properties and applications.
Structural and Functional Comparisons
Table 1: Key Properties of (3R,4S)-4-Fluorotetrahydrofuran-3-ol and Analogs
Key Observations:
Fluorine Substitution: The target compound and the triazolo-pyrimidinyl derivative both feature a fluorine atom at C4, enhancing their metabolic stability and binding affinity in biological systems. However, the triazolo-pyrimidinyl group in the latter compound expands its utility in nucleoside analog synthesis for antiviral or anticancer therapies. In contrast, the dimethyl analog lacks fluorine, reducing polarity and increasing lipophilicity, which may favor applications in material science or non-polar solvents.
Hydroxyl Group Reactivity :
- The hydroxyl group at C3 in all compounds enables hydrogen bonding and derivatization (e.g., glycosylation, esterification). However, steric hindrance varies:
- The benzylamino group in introduces bulkiness, limiting accessibility for certain reactions compared to the smaller -F and -CH₃ groups in other analogs.
Stereochemical Impact :
- The (3R,4S) configuration in the target compound and contrasts with the (3S,4S) stereochemistry in . Such differences influence enantioselectivity in catalysis and receptor binding, as seen in ’s comparison of stereoisomer binding modes .
Application-Specific Comparisons
- Pharmaceutical Potential: The triazolo-pyrimidinyl derivative is explicitly noted for its role in drug candidates and agrochemicals due to its high purity and complex functionalization . The target compound, with fewer substituents, may serve as a simpler scaffold for probing fluorine’s isolated effects.
- Chemical Synthesis: The dimethyl analog ’s lower molecular weight and non-polar substituents make it suitable for solvent applications or polymer precursors , whereas the benzylamino derivative is tailored for asymmetric synthesis .
- Safety and Handling :
- While safety data for the target compound are unavailable, analogs like and require stringent storage conditions (e.g., room temperature, inert atmospheres) to maintain stability, as inferred from their structural complexity .
Biological Activity
(3R,4S)-4-Fluorotetrahydrofuran-3-ol is a fluorinated organic compound that has garnered attention in various fields of biological research due to its potential activity as a pesticide intermediate and its interactions with biological systems. This article reviews the compound's biological activity, including its biochemical properties, cellular effects, molecular mechanisms, and relevant case studies.
The biochemical properties of this compound suggest significant interactions with enzymes and proteins. The compound exhibits the ability to form covalent bonds with active sites of enzymes, leading to modulation or inhibition of enzymatic activity. Such interactions are crucial for its role in biochemical pathways and suggest potential applications in pest control by targeting essential enzymes in pests.
Cellular Effects
The cellular effects of this compound vary depending on the cell type. Studies indicate that the compound can alter signaling pathways, impacting gene expression and cellular metabolism. For instance, it has been shown to modulate the activities of kinases and phosphatases, which are critical for phosphorylation events in cell signaling.
Table 1: Summary of Cellular Effects
Cell Type | Observed Effects |
---|---|
Neuronal Cells | Modulation of neurotransmitter release |
Cancer Cell Lines | Altered proliferation rates |
Immune Cells | Changes in cytokine production |
Molecular Mechanisms
At the molecular level, this compound interacts with various enzymes through both covalent and non-covalent interactions. The binding affinity to enzyme active sites is influenced by the presence of the fluorine atom, which enhances lipophilicity and membrane permeability . This characteristic is critical for its biological activity as it facilitates better interaction with target proteins.
Metabolic Pathways
The compound is metabolized primarily by cytochrome P450 enzymes, leading to various metabolites that may exhibit distinct biological activities. Understanding these metabolic pathways is essential for predicting the compound's behavior in biological systems and its potential therapeutic applications.
Dosage Effects in Animal Models
Research indicates that dosage significantly influences the biological activity of this compound in animal models. Low doses may exhibit minimal adverse effects, while higher doses can lead to toxicity. For example, toxicity studies have shown that doses exceeding certain thresholds result in significant physiological alterations.
Case Studies and Research Findings
Several studies have investigated the biological activity of this compound:
- Pesticide Development : As a pesticide intermediate, this compound has been evaluated for its efficacy against specific pests by targeting metabolic enzymes crucial for their survival.
- Cancer Research : In vitro studies have shown that this compound can inhibit cancer cell growth by inducing apoptosis through modulation of signaling pathways related to cell survival and proliferation .
- Neuropharmacology : The compound has been studied for its effects on neurotransmitter systems, suggesting potential applications in treating neurodegenerative diseases by enhancing synaptic function through enzyme modulation .
Q & A
Q. How can stereochemical purity of (3R,4S)-4-Fluorotetrahydrofuran-3-ol be ensured during synthesis?
Advanced Question
The stereochemical integrity of this compound is critical for its biological and chemical applications. To ensure high enantiomeric excess (ee):
- Chiral Auxiliaries : Use enantioselective catalysts (e.g., asymmetric hydrogenation catalysts) or chiral resolving agents during ring-closing reactions of tetrahydrofuran precursors .
- Analytical Validation : Employ chiral HPLC or polarimetry to confirm stereochemistry. Compare retention times with known standards or use Mosher ester derivatization for absolute configuration determination .
- Synthetic Route Optimization : Avoid harsh reaction conditions (e.g., high temperatures) that may induce racemization. Evidence from similar fluorinated tetrahydrofurans suggests that low-temperature nucleophilic fluorination preserves stereochemistry .
Q. What spectroscopic methods are most effective for characterizing the structure and purity of this compound?
Basic Question
A multi-technique approach is required:
- NMR Spectroscopy :
- Mass Spectrometry (HRMS) : Confirm molecular weight and isotopic patterns. ESI-MS is preferred for polar derivatives.
- IR Spectroscopy : Detect functional groups (e.g., -OH stretch at ~3200 cm⁻¹, C-F stretch at ~1100 cm⁻¹) .
Q. How does the fluorine substituent influence the compound’s reactivity in nucleophilic substitution reactions?
Advanced Question
Fluorine’s electronegativity and steric effects modulate reactivity:
- Electronic Effects : The electron-withdrawing nature of fluorine decreases electron density at C4, making it less susceptible to nucleophilic attack. However, adjacent hydroxyl groups (C3) may participate in hydrogen bonding, stabilizing transition states .
- Steric Hindrance : Fluorine’s small atomic radius minimizes steric interference, enabling selective functionalization at C3. Computational studies (DFT) on analogous compounds suggest that fluorine stabilizes partial charges during SN2 mechanisms .
- Experimental Validation : Compare reaction rates with non-fluorinated analogs (e.g., tetrahydrofuran-3-ol) using kinetic assays .
Q. What strategies mitigate decomposition of this compound under storage?
Basic Question
Stability challenges arise from hydroxyl and fluorine group interactions:
- Storage Conditions :
- Stabilizers : Add radical scavengers (e.g., BHT) to inhibit oxidative degradation. For aqueous solutions, buffer at pH 5–6 to avoid acid/base-catalyzed ring-opening .
Q. How can computational modeling predict the biological activity of this compound derivatives?
Advanced Question
Leverage in silico tools for rational drug design:
- Molecular Docking : Simulate binding to target enzymes (e.g., oxidoreductases) using software like AutoDock Vina. Fluorine’s role in hydrophobic interactions can be quantified via binding energy scores .
- QSAR Models : Train models on fluorinated tetrahydrofuran analogs to correlate substituent patterns with activity (e.g., IC₅₀ values). Include descriptors like logP, polar surface area, and H-bond donor count .
- MD Simulations : Assess conformational stability in biological membranes. Fluorine may enhance membrane permeability by reducing polarity .
Q. How to resolve contradictions in reported synthetic yields for this compound?
Advanced Question
Discrepancies often arise from reaction conditions or purification methods:
- Critical Variables :
- Reproducibility : Cross-validate results using alternative routes (e.g., enzymatic resolution for stereocontrol) .
Q. What in vitro assays are suitable for evaluating the compound’s enzyme inhibition potential?
Basic Question
Design assays tailored to fluorinated tetrahydrofurans:
- Kinetic Assays : Measure IC₅₀ via fluorogenic substrates (e.g., 4-methylumbelliferyl derivatives) for hydrolases. Monitor fluorescence quenching caused by fluorine’s electronegativity .
- Thermal Shift Assays : Detect target binding by measuring protein melting temperature () shifts using SYPRO Orange dye .
- Control Experiments : Include non-fluorinated analogs to isolate fluorine-specific effects .
Q. How does stereochemistry impact the compound’s pharmacokinetic properties?
Advanced Question
The (3R,4S) configuration influences ADME profiles:
- Absorption : The hydroxyl group enhances solubility, while fluorine improves lipid membrane penetration. Use Caco-2 cell monolayers to measure apparent permeability () .
- Metabolism : Stereochemistry affects susceptibility to cytochrome P450 enzymes. Perform liver microsome assays with NADPH cofactors to identify major metabolites .
- Excretion : Radiolabeled studies (e.g., ¹⁸F isotopes) can track renal clearance pathways .
Properties
IUPAC Name |
(3R,4S)-4-fluorooxolan-3-ol | |
---|---|---|
Source | PubChem | |
URL | https://pubchem.ncbi.nlm.nih.gov | |
Description | Data deposited in or computed by PubChem | |
InChI |
InChI=1S/C4H7FO2/c5-3-1-7-2-4(3)6/h3-4,6H,1-2H2/t3-,4+/m0/s1 | |
Source | PubChem | |
URL | https://pubchem.ncbi.nlm.nih.gov | |
Description | Data deposited in or computed by PubChem | |
InChI Key |
INQHWCYKASVEQT-IUYQGCFVSA-N | |
Source | PubChem | |
URL | https://pubchem.ncbi.nlm.nih.gov | |
Description | Data deposited in or computed by PubChem | |
Canonical SMILES |
C1C(C(CO1)F)O | |
Source | PubChem | |
URL | https://pubchem.ncbi.nlm.nih.gov | |
Description | Data deposited in or computed by PubChem | |
Isomeric SMILES |
C1[C@H]([C@H](CO1)F)O | |
Source | PubChem | |
URL | https://pubchem.ncbi.nlm.nih.gov | |
Description | Data deposited in or computed by PubChem | |
Molecular Formula |
C4H7FO2 | |
Source | PubChem | |
URL | https://pubchem.ncbi.nlm.nih.gov | |
Description | Data deposited in or computed by PubChem | |
Molecular Weight |
106.10 g/mol | |
Source | PubChem | |
URL | https://pubchem.ncbi.nlm.nih.gov | |
Description | Data deposited in or computed by PubChem | |
Retrosynthesis Analysis
AI-Powered Synthesis Planning: Our tool employs the Template_relevance Pistachio, Template_relevance Bkms_metabolic, Template_relevance Pistachio_ringbreaker, Template_relevance Reaxys, Template_relevance Reaxys_biocatalysis model, leveraging a vast database of chemical reactions to predict feasible synthetic routes.
One-Step Synthesis Focus: Specifically designed for one-step synthesis, it provides concise and direct routes for your target compounds, streamlining the synthesis process.
Accurate Predictions: Utilizing the extensive PISTACHIO, BKMS_METABOLIC, PISTACHIO_RINGBREAKER, REAXYS, REAXYS_BIOCATALYSIS database, our tool offers high-accuracy predictions, reflecting the latest in chemical research and data.
Strategy Settings
Precursor scoring | Relevance Heuristic |
---|---|
Min. plausibility | 0.01 |
Model | Template_relevance |
Template Set | Pistachio/Bkms_metabolic/Pistachio_ringbreaker/Reaxys/Reaxys_biocatalysis |
Top-N result to add to graph | 6 |
Feasible Synthetic Routes
Disclaimer and Information on In-Vitro Research Products
Please be aware that all articles and product information presented on BenchChem are intended solely for informational purposes. The products available for purchase on BenchChem are specifically designed for in-vitro studies, which are conducted outside of living organisms. In-vitro studies, derived from the Latin term "in glass," involve experiments performed in controlled laboratory settings using cells or tissues. It is important to note that these products are not categorized as medicines or drugs, and they have not received approval from the FDA for the prevention, treatment, or cure of any medical condition, ailment, or disease. We must emphasize that any form of bodily introduction of these products into humans or animals is strictly prohibited by law. It is essential to adhere to these guidelines to ensure compliance with legal and ethical standards in research and experimentation.