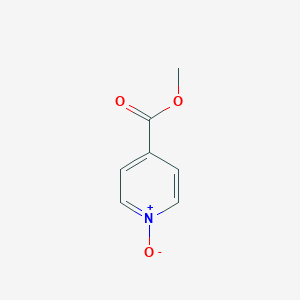
Methylisonicotinate-N-oxide
Overview
Description
Methylisonicotinate-N-oxide is a chemical compound with the molecular formula C7H7NO3 and a molecular weight of 153.14 g/mol . It is also known by its IUPAC name, methyl 1-oxidopyridin-1-ium-4-carboxylate . This compound is characterized by the presence of a pyridine ring substituted with a methoxycarbonyl group and an N-oxide functional group.
Preparation Methods
The synthesis of Methylisonicotinate-N-oxide can be achieved through various methods. One common synthetic route involves the use of copper (I) iodide and N,N-Dimethylcarbamoyl chloride in acetonitrile at 90°C for 7 hours. Another method utilizes zinc cyanide instead of sodium cyanide, with the reaction taking place at 80°C for 5 hours. These methods highlight the versatility of the compound’s synthesis, allowing for different reaction conditions and reagents to be employed.
Chemical Reactions Analysis
Methylisonicotinate-N-oxide undergoes various types of chemical reactions, including oxidation, reduction, and substitution reactions. Common reagents used in these reactions include oxidizing agents like hydrogen peroxide and reducing agents such as sodium borohydride. The compound can also participate in substitution reactions with nucleophiles, leading to the formation of various derivatives . Major products formed from these reactions depend on the specific reagents and conditions used, but they often include substituted pyridine derivatives and other functionalized compounds.
Scientific Research Applications
Methylisonicotinate-N-oxide has a wide range of scientific research applications. In chemistry, it is used as a building block for the synthesis of more complex molecules and as a reagent in various organic reactions . In biology and medicine, the compound has been studied for its potential therapeutic properties, including its ability to act as an enzyme inhibitor and its potential use in drug development . Additionally, in the industrial sector, this compound is used in the production of specialty chemicals and as an intermediate in the synthesis of agrochemicals and pharmaceuticals .
Mechanism of Action
The mechanism of action of Methylisonicotinate-N-oxide involves its ability to act as a mild Lewis base, activating certain Lewis acidic parts of molecules and increasing the reactivity of its nucleophilic part towards various reactions with electrophiles . This activation mechanism allows the compound to participate in a wide range of chemical reactions, making it a valuable reagent in organic synthesis. The molecular targets and pathways involved in its action depend on the specific application and the nature of the reaction it is involved in.
Comparison with Similar Compounds
Methylisonicotinate-N-oxide can be compared with other similar compounds, such as pyridine N-oxide, 4-methoxypyridine N-oxide, and 4-pyridone . These compounds share structural similarities, including the presence of a pyridine ring and an N-oxide functional group. this compound is unique due to the presence of the methoxycarbonyl group, which imparts distinct chemical properties and reactivity. This uniqueness makes it a valuable compound for specific applications in research and industry.
Properties
IUPAC Name |
methyl 1-oxidopyridin-1-ium-4-carboxylate | |
---|---|---|
Source | PubChem | |
URL | https://pubchem.ncbi.nlm.nih.gov | |
Description | Data deposited in or computed by PubChem | |
InChI |
InChI=1S/C7H7NO3/c1-11-7(9)6-2-4-8(10)5-3-6/h2-5H,1H3 | |
Source | PubChem | |
URL | https://pubchem.ncbi.nlm.nih.gov | |
Description | Data deposited in or computed by PubChem | |
InChI Key |
XSGNXRNOZMUURC-UHFFFAOYSA-N | |
Source | PubChem | |
URL | https://pubchem.ncbi.nlm.nih.gov | |
Description | Data deposited in or computed by PubChem | |
Canonical SMILES |
COC(=O)C1=CC=[N+](C=C1)[O-] | |
Source | PubChem | |
URL | https://pubchem.ncbi.nlm.nih.gov | |
Description | Data deposited in or computed by PubChem | |
Molecular Formula |
C7H7NO3 | |
Source | PubChem | |
URL | https://pubchem.ncbi.nlm.nih.gov | |
Description | Data deposited in or computed by PubChem | |
DSSTOX Substance ID |
DTXSID60284844 | |
Record name | Methyl 1-oxo-1lambda~5~-pyridine-4-carboxylate | |
Source | EPA DSSTox | |
URL | https://comptox.epa.gov/dashboard/DTXSID60284844 | |
Description | DSSTox provides a high quality public chemistry resource for supporting improved predictive toxicology. | |
Molecular Weight |
153.14 g/mol | |
Source | PubChem | |
URL | https://pubchem.ncbi.nlm.nih.gov | |
Description | Data deposited in or computed by PubChem | |
CAS No. |
3783-38-8 | |
Record name | 3783-38-8 | |
Source | DTP/NCI | |
URL | https://dtp.cancer.gov/dtpstandard/servlet/dwindex?searchtype=NSC&outputformat=html&searchlist=39284 | |
Description | The NCI Development Therapeutics Program (DTP) provides services and resources to the academic and private-sector research communities worldwide to facilitate the discovery and development of new cancer therapeutic agents. | |
Explanation | Unless otherwise indicated, all text within NCI products is free of copyright and may be reused without our permission. Credit the National Cancer Institute as the source. | |
Record name | Methyl 1-oxo-1lambda~5~-pyridine-4-carboxylate | |
Source | EPA DSSTox | |
URL | https://comptox.epa.gov/dashboard/DTXSID60284844 | |
Description | DSSTox provides a high quality public chemistry resource for supporting improved predictive toxicology. | |
Record name | 3783-38-8 | |
Source | European Chemicals Agency (ECHA) | |
URL | https://echa.europa.eu/information-on-chemicals | |
Description | The European Chemicals Agency (ECHA) is an agency of the European Union which is the driving force among regulatory authorities in implementing the EU's groundbreaking chemicals legislation for the benefit of human health and the environment as well as for innovation and competitiveness. | |
Explanation | Use of the information, documents and data from the ECHA website is subject to the terms and conditions of this Legal Notice, and subject to other binding limitations provided for under applicable law, the information, documents and data made available on the ECHA website may be reproduced, distributed and/or used, totally or in part, for non-commercial purposes provided that ECHA is acknowledged as the source: "Source: European Chemicals Agency, http://echa.europa.eu/". Such acknowledgement must be included in each copy of the material. ECHA permits and encourages organisations and individuals to create links to the ECHA website under the following cumulative conditions: Links can only be made to webpages that provide a link to the Legal Notice page. | |
Synthesis routes and methods I
Procedure details
Synthesis routes and methods II
Procedure details
Retrosynthesis Analysis
AI-Powered Synthesis Planning: Our tool employs the Template_relevance Pistachio, Template_relevance Bkms_metabolic, Template_relevance Pistachio_ringbreaker, Template_relevance Reaxys, Template_relevance Reaxys_biocatalysis model, leveraging a vast database of chemical reactions to predict feasible synthetic routes.
One-Step Synthesis Focus: Specifically designed for one-step synthesis, it provides concise and direct routes for your target compounds, streamlining the synthesis process.
Accurate Predictions: Utilizing the extensive PISTACHIO, BKMS_METABOLIC, PISTACHIO_RINGBREAKER, REAXYS, REAXYS_BIOCATALYSIS database, our tool offers high-accuracy predictions, reflecting the latest in chemical research and data.
Strategy Settings
Precursor scoring | Relevance Heuristic |
---|---|
Min. plausibility | 0.01 |
Model | Template_relevance |
Template Set | Pistachio/Bkms_metabolic/Pistachio_ringbreaker/Reaxys/Reaxys_biocatalysis |
Top-N result to add to graph | 6 |
Feasible Synthetic Routes
Disclaimer and Information on In-Vitro Research Products
Please be aware that all articles and product information presented on BenchChem are intended solely for informational purposes. The products available for purchase on BenchChem are specifically designed for in-vitro studies, which are conducted outside of living organisms. In-vitro studies, derived from the Latin term "in glass," involve experiments performed in controlled laboratory settings using cells or tissues. It is important to note that these products are not categorized as medicines or drugs, and they have not received approval from the FDA for the prevention, treatment, or cure of any medical condition, ailment, or disease. We must emphasize that any form of bodily introduction of these products into humans or animals is strictly prohibited by law. It is essential to adhere to these guidelines to ensure compliance with legal and ethical standards in research and experimentation.