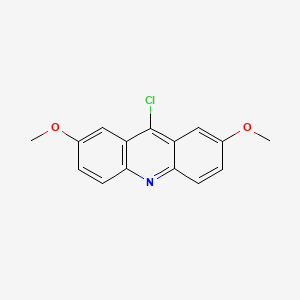
9-Chloro-2,7-dimethoxyacridine
Overview
Description
9-Chloro-2,7-dimethoxyacridine is a heterocyclic aromatic compound belonging to the acridine family. Acridines are known for their diverse applications in various fields, including medicine, biology, and chemistry. This compound, characterized by its chlorine and methoxy substituents, exhibits unique chemical properties that make it valuable for research and industrial applications .
Preparation Methods
Synthetic Routes and Reaction Conditions: The synthesis of 9-Chloro-2,7-dimethoxyacridine typically involves the chlorination of 2,7-dimethoxyacridine. One common method includes the reaction of 2,7-dimethoxyacridine with thionyl chloride (SOCl2) or phosphorus pentachloride (PCl5) under controlled conditions . The reaction is usually carried out in an inert solvent such as dichloromethane or chloroform, and the temperature is maintained at a moderate level to ensure the selective chlorination at the 9-position.
Industrial Production Methods: Industrial production of this compound follows similar synthetic routes but on a larger scale. The process involves the use of large reactors and precise control of reaction parameters to achieve high yields and purity. The product is then purified using techniques such as recrystallization or column chromatography .
Chemical Reactions Analysis
Types of Reactions: 9-Chloro-2,7-dimethoxyacridine undergoes various chemical reactions, including:
Substitution Reactions: The chlorine atom at the 9-position can be substituted with other nucleophiles, such as amines or thiols, under appropriate conditions.
Oxidation and Reduction Reactions: The compound can be oxidized or reduced to form different derivatives, which can be useful in various applications.
Common Reagents and Conditions:
Substitution Reactions: Common reagents include amines, thiols, and other nucleophiles.
Oxidation and Reduction Reactions: Oxidizing agents like potassium permanganate (KMnO4) or reducing agents like sodium borohydride (NaBH4) are used under controlled conditions.
Major Products Formed:
Substitution Products: Various substituted acridines depending on the nucleophile used.
Oxidation and Reduction Products: Different oxidized or reduced forms of the original compound.
Scientific Research Applications
9-Chloro-2,7-dimethoxyacridine has a wide range of applications in scientific research:
Mechanism of Action
The mechanism of action of 9-Chloro-2,7-dimethoxyacridine involves its interaction with various molecular targets. The compound can intercalate into DNA, disrupting the normal function of the genetic material and leading to cell death. This property makes it a potential candidate for anticancer and antimicrobial therapies . Additionally, it can inhibit certain enzymes, further contributing to its biological activity .
Comparison with Similar Compounds
- 9-Bromo-2,7-dimethoxyacridine
- 9-Fluoro-2,7-dimethoxyacridine
- 9-Amino-2,7-dimethoxyacridine
Comparison: Compared to its analogs, 9-Chloro-2,7-dimethoxyacridine exhibits unique properties due to the presence of the chlorine atom. This substitution affects its reactivity and interaction with biological targets, making it distinct in its applications . For instance, the chlorine atom can be easily substituted, allowing for the synthesis of a wide range of derivatives with potential therapeutic applications .
Properties
IUPAC Name |
9-chloro-2,7-dimethoxyacridine | |
---|---|---|
Source | PubChem | |
URL | https://pubchem.ncbi.nlm.nih.gov | |
Description | Data deposited in or computed by PubChem | |
InChI |
InChI=1S/C15H12ClNO2/c1-18-9-3-5-13-11(7-9)15(16)12-8-10(19-2)4-6-14(12)17-13/h3-8H,1-2H3 | |
Source | PubChem | |
URL | https://pubchem.ncbi.nlm.nih.gov | |
Description | Data deposited in or computed by PubChem | |
InChI Key |
BXRKDNPFLFXVAZ-UHFFFAOYSA-N | |
Source | PubChem | |
URL | https://pubchem.ncbi.nlm.nih.gov | |
Description | Data deposited in or computed by PubChem | |
Canonical SMILES |
COC1=CC2=C(C=C1)N=C3C=CC(=CC3=C2Cl)OC | |
Source | PubChem | |
URL | https://pubchem.ncbi.nlm.nih.gov | |
Description | Data deposited in or computed by PubChem | |
Molecular Formula |
C15H12ClNO2 | |
Source | PubChem | |
URL | https://pubchem.ncbi.nlm.nih.gov | |
Description | Data deposited in or computed by PubChem | |
DSSTOX Substance ID |
DTXSID80856603 | |
Record name | 9-Chloro-2,7-dimethoxyacridine | |
Source | EPA DSSTox | |
URL | https://comptox.epa.gov/dashboard/DTXSID80856603 | |
Description | DSSTox provides a high quality public chemistry resource for supporting improved predictive toxicology. | |
Molecular Weight |
273.71 g/mol | |
Source | PubChem | |
URL | https://pubchem.ncbi.nlm.nih.gov | |
Description | Data deposited in or computed by PubChem | |
CAS No. |
6526-92-7 | |
Record name | 9-Chloro-2,7-dimethoxyacridine | |
Source | EPA DSSTox | |
URL | https://comptox.epa.gov/dashboard/DTXSID80856603 | |
Description | DSSTox provides a high quality public chemistry resource for supporting improved predictive toxicology. | |
Retrosynthesis Analysis
AI-Powered Synthesis Planning: Our tool employs the Template_relevance Pistachio, Template_relevance Bkms_metabolic, Template_relevance Pistachio_ringbreaker, Template_relevance Reaxys, Template_relevance Reaxys_biocatalysis model, leveraging a vast database of chemical reactions to predict feasible synthetic routes.
One-Step Synthesis Focus: Specifically designed for one-step synthesis, it provides concise and direct routes for your target compounds, streamlining the synthesis process.
Accurate Predictions: Utilizing the extensive PISTACHIO, BKMS_METABOLIC, PISTACHIO_RINGBREAKER, REAXYS, REAXYS_BIOCATALYSIS database, our tool offers high-accuracy predictions, reflecting the latest in chemical research and data.
Strategy Settings
Precursor scoring | Relevance Heuristic |
---|---|
Min. plausibility | 0.01 |
Model | Template_relevance |
Template Set | Pistachio/Bkms_metabolic/Pistachio_ringbreaker/Reaxys/Reaxys_biocatalysis |
Top-N result to add to graph | 6 |
Feasible Synthetic Routes
Disclaimer and Information on In-Vitro Research Products
Please be aware that all articles and product information presented on BenchChem are intended solely for informational purposes. The products available for purchase on BenchChem are specifically designed for in-vitro studies, which are conducted outside of living organisms. In-vitro studies, derived from the Latin term "in glass," involve experiments performed in controlled laboratory settings using cells or tissues. It is important to note that these products are not categorized as medicines or drugs, and they have not received approval from the FDA for the prevention, treatment, or cure of any medical condition, ailment, or disease. We must emphasize that any form of bodily introduction of these products into humans or animals is strictly prohibited by law. It is essential to adhere to these guidelines to ensure compliance with legal and ethical standards in research and experimentation.