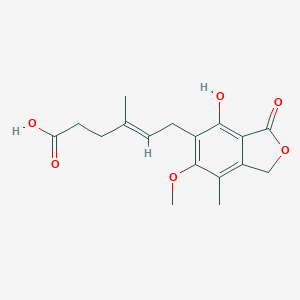
Mycophenolic acid
Overview
Description
Mycophenolic acid is a potent immunosuppressant agent derived from the fungus Penicillium stoloniferum. It is primarily used to prevent organ transplant rejection and has shown antibacterial, antifungal, and antiviral properties . This compound works by inhibiting de novo purine biosynthesis, which is essential for the proliferation of lymphocytes .
Biochemical Analysis
Biochemical Properties
Mycophenolic acid plays a significant role in biochemical reactions by inhibiting inosine monophosphate dehydrogenase (IMPDH), an enzyme critical for the de novo synthesis of guanine nucleotides . This inhibition leads to a decrease in guanosine triphosphate (GTP) levels, which are essential for DNA and RNA synthesis. This compound interacts with various biomolecules, including IMPDH, and prevents the conversion of inosine monophosphate (IMP) to xanthosine monophosphate (XMP), thereby reducing the availability of guanine nucleotides .
Cellular Effects
This compound affects various types of cells and cellular processes. It has a cytostatic effect on T and B lymphocytes, inhibiting their proliferation . This compound also suppresses antibody formation by B lymphocytes and prevents the glycosylation of lymphocyte and monocyte glycoproteins involved in intercellular adhesion to endothelial cells . Additionally, this compound influences cell signaling pathways, gene expression, and cellular metabolism by reducing the availability of guanine nucleotides .
Molecular Mechanism
The molecular mechanism of this compound involves the inhibition of inosine monophosphate dehydrogenase (IMPDH), which is crucial for the synthesis of guanine nucleotides . By binding to IMPDH, this compound prevents the conversion of inosine monophosphate (IMP) to xanthosine monophosphate (XMP), leading to a decrease in guanosine triphosphate (GTP) levels . This reduction in GTP levels affects DNA and RNA synthesis, ultimately inhibiting the proliferation of lymphocytes .
Temporal Effects in Laboratory Settings
In laboratory settings, the effects of this compound change over time. The compound is rapidly hydrolyzed to its active form, this compound, which has a half-life of approximately 17.9 hours . Over time, this compound can degrade, leading to a decrease in its immunosuppressive effects. Long-term studies have shown that this compound can cause gastrointestinal adverse effects, including diarrhea and nausea .
Dosage Effects in Animal Models
In animal models, the effects of this compound vary with different dosages. At lower doses, this compound effectively inhibits lymphocyte proliferation without causing significant adverse effects . At higher doses, this compound can cause toxic effects, including gastrointestinal disturbances and bone marrow suppression . Threshold effects have been observed, with higher doses leading to more pronounced immunosuppressive effects .
Metabolic Pathways
This compound is involved in several metabolic pathways, primarily through its inhibition of inosine monophosphate dehydrogenase (IMPDH) . This inhibition affects the de novo synthesis of guanine nucleotides, leading to a decrease in guanosine triphosphate (GTP) levels . This compound is metabolized in the liver by glucuronidation, forming this compound glucuronide (MPAG), which is then excreted in the urine .
Transport and Distribution
This compound is transported and distributed within cells and tissues through various mechanisms. It is highly protein-bound in the blood, with up to 97% of the compound bound to albumin . This compound is absorbed in the small intestine and distributed to various tissues, including the liver, where it undergoes metabolism . The compound’s distribution is influenced by its binding to plasma proteins and its ability to cross cell membranes .
Subcellular Localization
The subcellular localization of this compound involves its distribution to various cellular compartments. This compound biosynthetic enzymes are compartmentalized, with the polyketide synthase MpaC’ located in the cytosol, the prenyltransferase MpaA’ associated with the Golgi apparatus, and the oxygenase MpaB’ bound to the endoplasmic reticulum . This compartmentalization is essential for the proper functioning of the biosynthetic pathway and the compound’s immunosuppressive effects .
Preparation Methods
Synthetic Routes and Reaction Conditions
Mycophenolic acid can be synthesized through microbial fermentation using various species of Penicillium, including Penicillium brevicompactum . The production involves submerged fermentation, where the yield can be optimized by adjusting factors such as carbon and nitrogen sources, phosphate sources, temperature, and pH . The fermentation process typically lasts around 180 hours, and the product is purified using high-performance liquid chromatography .
Industrial Production Methods
Industrial production of this compound involves large-scale fermentation in stirred fermenters. The process includes optimizing the fermentation medium and conditions to maximize yield. The sodium salt of this compound and mycophenolate mofetil, a derivative, are also produced for clinical use .
Chemical Reactions Analysis
Types of Reactions
Mycophenolic acid undergoes various chemical reactions, including oxidation, reduction, and substitution. It is known to inhibit inosine monophosphate dehydrogenase, which is crucial for guanosine synthesis in lymphocytes .
Common Reagents and Conditions
Common reagents used in the reactions involving this compound include oxidizing agents, reducing agents, and various solvents. The conditions for these reactions are typically mild to moderate temperatures and neutral to slightly acidic pH .
Major Products Formed
The major products formed from the reactions of this compound include its sodium salt and mycophenolate mofetil. These derivatives are used clinically to prevent organ transplant rejection .
Scientific Research Applications
Mycophenolic acid has a wide range of scientific research applications:
Mechanism of Action
Mycophenolic acid exerts its effects by selectively inhibiting inosine monophosphate dehydrogenase, an enzyme crucial for the de novo synthesis of guanosine nucleotides . This inhibition leads to a depletion of guanosine nucleotides, which are essential for the proliferation of T and B lymphocytes . As a result, this compound suppresses cell-mediated immune responses and antibody formation .
Comparison with Similar Compounds
Mycophenolic acid is unique in its potent immunosuppressive properties and its ability to inhibit inosine monophosphate dehydrogenase. Similar compounds include:
Azathioprine: Another immunosuppressant used to prevent organ transplant rejection, but with a different mechanism of action.
Methotrexate: Used to treat autoimmune diseases and certain cancers, it inhibits dihydrofolate reductase, affecting purine and pyrimidine synthesis.
Cyclosporine: An immunosuppressant that inhibits calcineurin, preventing the activation of T-cells.
This compound stands out due to its specific inhibition of inosine monophosphate dehydrogenase and its effectiveness in preventing organ transplant rejection .
Properties
IUPAC Name |
6-(4-hydroxy-6-methoxy-7-methyl-3-oxo-1H-2-benzofuran-5-yl)-4-methylhex-4-enoic acid | |
---|---|---|
Details | Computed by Lexichem TK 2.7.0 (PubChem release 2021.05.07) | |
Source | PubChem | |
URL | https://pubchem.ncbi.nlm.nih.gov | |
Description | Data deposited in or computed by PubChem | |
InChI |
InChI=1S/C17H20O6/c1-9(5-7-13(18)19)4-6-11-15(20)14-12(8-23-17(14)21)10(2)16(11)22-3/h4,20H,5-8H2,1-3H3,(H,18,19) | |
Details | Computed by InChI 1.0.6 (PubChem release 2021.05.07) | |
Source | PubChem | |
URL | https://pubchem.ncbi.nlm.nih.gov | |
Description | Data deposited in or computed by PubChem | |
InChI Key |
HPNSFSBZBAHARI-UHFFFAOYSA-N | |
Details | Computed by InChI 1.0.6 (PubChem release 2021.05.07) | |
Source | PubChem | |
URL | https://pubchem.ncbi.nlm.nih.gov | |
Description | Data deposited in or computed by PubChem | |
Canonical SMILES |
CC1=C2COC(=O)C2=C(C(=C1OC)CC=C(C)CCC(=O)O)O | |
Details | Computed by OEChem 2.3.0 (PubChem release 2021.05.07) | |
Source | PubChem | |
URL | https://pubchem.ncbi.nlm.nih.gov | |
Description | Data deposited in or computed by PubChem | |
Molecular Formula |
C17H20O6 | |
Details | Computed by PubChem 2.1 (PubChem release 2021.05.07) | |
Source | PubChem | |
URL | https://pubchem.ncbi.nlm.nih.gov | |
Description | Data deposited in or computed by PubChem | |
DSSTOX Substance ID |
DTXSID70860315 | |
Record name | 6-(4-Hydroxy-6-methoxy-7-methyl-3-oxo-1,3-dihydro-2-benzofuran-5-yl)-4-methylhex-4-enoic acid | |
Source | EPA DSSTox | |
URL | https://comptox.epa.gov/dashboard/DTXSID70860315 | |
Description | DSSTox provides a high quality public chemistry resource for supporting improved predictive toxicology. | |
Molecular Weight |
320.3 g/mol | |
Details | Computed by PubChem 2.1 (PubChem release 2021.05.07) | |
Source | PubChem | |
URL | https://pubchem.ncbi.nlm.nih.gov | |
Description | Data deposited in or computed by PubChem | |
CAS No. |
483-60-3 | |
Record name | 6-(1,3-Dihydro-4-hydroxy-6-methoxy-7-methyl-3-oxo-5-isobenzofuranyl)-4-methyl-4-hexenoic acid | |
Source | CAS Common Chemistry | |
URL | https://commonchemistry.cas.org/detail?cas_rn=483-60-3 | |
Description | CAS Common Chemistry is an open community resource for accessing chemical information. Nearly 500,000 chemical substances from CAS REGISTRY cover areas of community interest, including common and frequently regulated chemicals, and those relevant to high school and undergraduate chemistry classes. This chemical information, curated by our expert scientists, is provided in alignment with our mission as a division of the American Chemical Society. | |
Explanation | The data from CAS Common Chemistry is provided under a CC-BY-NC 4.0 license, unless otherwise stated. | |
Retrosynthesis Analysis
AI-Powered Synthesis Planning: Our tool employs the Template_relevance Pistachio, Template_relevance Bkms_metabolic, Template_relevance Pistachio_ringbreaker, Template_relevance Reaxys, Template_relevance Reaxys_biocatalysis model, leveraging a vast database of chemical reactions to predict feasible synthetic routes.
One-Step Synthesis Focus: Specifically designed for one-step synthesis, it provides concise and direct routes for your target compounds, streamlining the synthesis process.
Accurate Predictions: Utilizing the extensive PISTACHIO, BKMS_METABOLIC, PISTACHIO_RINGBREAKER, REAXYS, REAXYS_BIOCATALYSIS database, our tool offers high-accuracy predictions, reflecting the latest in chemical research and data.
Strategy Settings
Precursor scoring | Relevance Heuristic |
---|---|
Min. plausibility | 0.01 |
Model | Template_relevance |
Template Set | Pistachio/Bkms_metabolic/Pistachio_ringbreaker/Reaxys/Reaxys_biocatalysis |
Top-N result to add to graph | 6 |
Feasible Synthetic Routes
Q1: What is the primary mechanism of action of MPA?
A1: MPA is a potent, selective, non-competitive, and reversible inhibitor of inosine monophosphate dehydrogenase (IMPDH) [, ]. IMPDH catalyzes the rate-limiting step in the de novo synthesis of guanine nucleotides.
Q2: How does MPA's inhibition of IMPDH lead to immunosuppression?
A2: By inhibiting IMPDH, MPA depletes intracellular guanine nucleotide pools, particularly in lymphocytes [, ]. Lymphocytes rely heavily on the de novo pathway for guanine nucleotide synthesis, making them particularly susceptible to MPA's effects. This depletion inhibits lymphocyte proliferation and function, ultimately leading to immunosuppression.
Q3: Does MPA affect other cell types besides lymphocytes?
A3: While MPA primarily targets lymphocytes, it can impact other cell types if they rely heavily on the de novo pathway for guanine nucleotide synthesis []. One study found that MPA altered endothelial cell nucleotide levels, leading to changes in membrane glycosylation and E-selectin expression [].
Q4: What is the molecular formula and weight of MPA?
A4: The molecular formula for MPA is C17H20O6, and its molecular weight is 320.34 g/mol [, ].
Q5: Is there information available about the spectroscopic data for MPA?
A5: Unfortunately, the provided abstracts do not offer detailed spectroscopic data for MPA. Further research in spectroscopic databases or full-text articles is recommended.
Q6: How stable is MPA under various storage conditions?
A6: One study investigating an Emit assay for MPA found that samples were stable under various storage conditions, although storage at -20°C is recommended for periods longer than one day []. Specific stability data under varying pH, temperature, and light exposure would require further investigation.
Q7: What is the active metabolite of mycophenolate mofetil (MMF)?
A7: MMF is rapidly hydrolyzed to MPA, its pharmacologically active metabolite, after oral administration [, ].
Q8: How is MPA metabolized in the body?
A8: MPA is primarily metabolized by glucuronidation to its inactive metabolite, mycophenolic acid glucuronide (MPAG), and a lesser extent to its acyl glucuronide (AcMPAG) [, ].
Q9: What enzyme is responsible for the deglucuronidation of AcMPAG in the liver?
A9: Research has identified α/β hydrolase domain containing 10 (ABHD10) as the enzyme responsible for AcMPAG deglucuronidation in the human liver []. This enzyme could potentially influence the incidence of MPA-induced immunotoxicity associated with AcMPAG.
Q10: Does the co-administration of other immunosuppressants affect MPA pharmacokinetics?
A10: Yes, several studies have shown that co-administration of other immunosuppressants, particularly ciclosporin and sirolimus, can significantly alter the pharmacokinetics of MPA [, , ]. For instance, patients taking MMF with sirolimus experience higher MPA exposure and lower MPA metabolite exposure than those treated with MMF and ciclosporin [].
Q11: Does pantoprazole affect the bioavailability of MMF and enteric-coated mycophenolate sodium (EC-MPS)?
A11: Research indicates that while pantoprazole significantly lowers MPA exposure following MMF administration, it does not alter the pharmacokinetics of EC-MPS []. This differential effect could be attributed to the different formulations and absorption mechanisms of the two drugs.
Q12: Is there a correlation between MPA exposure and clinical outcomes in transplant recipients?
A12: Yes, studies have shown that MPA exposure, often measured as the area under the concentration-time curve (AUC), is correlated with both the risk of acute rejection and the occurrence of chronic lung allograft dysfunction in transplant recipients [, ].
Q13: How does MPA exposure vary among patients?
A13: MPA exhibits significant interpatient pharmacokinetic variability, influenced by factors such as race, sex, concurrent medications, and enterohepatic circulation [, ].
Q14: Can limited sampling strategies accurately estimate MPA exposure?
A14: Several studies have explored limited sampling strategies (LSS) to estimate MPA AUC [, , ]. While some LSS models show promise, their accuracy can vary depending on the patient population and the specific time points used for sampling.
Q15: Are there any known toxicities associated with MPA?
A15: MPA has been associated with hematologic abnormalities, viral infections, and an increased risk of certain cancers, particularly in long-term use []. Close monitoring of patients on MPA therapy is crucial to manage potential adverse effects.
Q16: Are there any specific drug delivery strategies being explored for MPA?
A16: While the provided abstracts do not delve into specific drug delivery strategies for MPA, research in this area could focus on improving targeted delivery to lymphocytes or reducing systemic exposure to minimize side effects.
Q17: What analytical methods are commonly used to measure MPA concentrations?
A17: High-performance liquid chromatography (HPLC) with UV or fluorescence detection and enzyme immunoassays are commonly used to measure MPA concentrations in biological samples [, , , ].
Q18: What considerations are important for developing an analytical method for MPA?
A18: Key considerations include sensitivity, specificity (especially regarding MPA metabolites like MPAG and AcMPAG), linearity, accuracy, precision, and the ability to process a high volume of samples [].
Q19: Can resistance to MPA develop?
A19: Yes, resistance to MPA can occur. One study found that resistant neuroblastoma cells exhibited a 5.4-fold increase in IMPDH activity compared to sensitive cells, suggesting a potential resistance mechanism [].
Q20: What are the alternatives to MPA in immunosuppressive therapy?
A20: Other immunosuppressive agents used in transplantation include calcineurin inhibitors (e.g., tacrolimus, cyclosporine), mTOR inhibitors (e.g., sirolimus, everolimus), and anti-thymocyte globulins [, ]. The choice of immunosuppressive regimen depends on various factors, including the type of transplant, patient characteristics, and risk factors.
Disclaimer and Information on In-Vitro Research Products
Please be aware that all articles and product information presented on BenchChem are intended solely for informational purposes. The products available for purchase on BenchChem are specifically designed for in-vitro studies, which are conducted outside of living organisms. In-vitro studies, derived from the Latin term "in glass," involve experiments performed in controlled laboratory settings using cells or tissues. It is important to note that these products are not categorized as medicines or drugs, and they have not received approval from the FDA for the prevention, treatment, or cure of any medical condition, ailment, or disease. We must emphasize that any form of bodily introduction of these products into humans or animals is strictly prohibited by law. It is essential to adhere to these guidelines to ensure compliance with legal and ethical standards in research and experimentation.