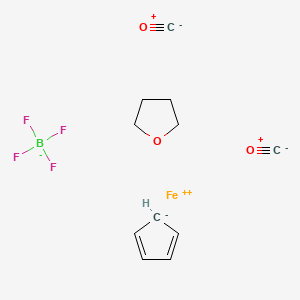
Cyclopentadienyldicarbonyl(tetrahydrofuran)iron(II) tetrafluoroborate
Overview
Description
Cyclopentadienyldicarbonyl(tetrahydrofuran)iron(II) tetrafluoroborate is a useful research compound. Its molecular formula is C11H13BF4FeO3 and its molecular weight is 335.87 g/mol. The purity is usually 95%.
The exact mass of the compound this compound is unknown and the complexity rating of the compound is unknown. The United Nations designated GHS hazard class pictogram is Irritant, and the GHS signal word is WarningThe storage condition is unknown. Please store according to label instructions upon receipt of goods.
BenchChem offers high-quality this compound suitable for many research applications. Different packaging options are available to accommodate customers' requirements. Please inquire for more information about this compound including the price, delivery time, and more detailed information at info@benchchem.com.
Mechanism of Action
Target of Action
Cyclopentadienyldicarbonyl(tetrahydrofuran)iron(II) tetrafluoroborate, also known as Carbon monoxide;cyclopenta-1,3-diene;iron(2+);oxolane;tetrafluoroborate, primarily targets chemical reactions as a catalyst . It is used in various Lewis acid-catalyzed reactions .
Mode of Action
This compound interacts with its targets by facilitating and accelerating chemical reactions. It does so by reducing the activation energy required for the reactions, thereby increasing the rate of these reactions .
Biochemical Pathways
The compound is involved in several biochemical pathways, including:
- The preparation of β-keto esters via Lewis acid-catalyzed addition of Ethyl diazoacetate to arylaldehydes .
- Iron mediated nitrenoid transfer .
- Lewis acid catalyzed aziridination, cyclopropanation, and insertion reaction with diazo compounds .
- Diels-Alder reaction of dienes with enones .
Pharmacokinetics
Its effectiveness can be influenced by factors such as concentration, temperature, and the presence of other substances in the reaction environment .
Result of Action
The primary result of the compound’s action is the facilitation of chemical reactions, leading to the production of desired compounds more efficiently and often with better yields .
Action Environment
The action, efficacy, and stability of the compound can be influenced by various environmental factors. For instance, temperature can affect the rate of the catalyzed reactions. Additionally, the presence of impurities or other substances can potentially interfere with the compound’s catalytic activity .
Properties
IUPAC Name |
carbon monoxide;cyclopenta-1,3-diene;iron(2+);oxolane;tetrafluoroborate | |
---|---|---|
Source | PubChem | |
URL | https://pubchem.ncbi.nlm.nih.gov | |
Description | Data deposited in or computed by PubChem | |
InChI |
InChI=1S/C5H5.C4H8O.2CO.BF4.Fe/c2*1-2-4-5-3-1;2*1-2;2-1(3,4)5;/h1-5H;1-4H2;;;;/q-1;;;;-1;+2 | |
Source | PubChem | |
URL | https://pubchem.ncbi.nlm.nih.gov | |
Description | Data deposited in or computed by PubChem | |
InChI Key |
QGGVUGKPZSNWHP-UHFFFAOYSA-N | |
Source | PubChem | |
URL | https://pubchem.ncbi.nlm.nih.gov | |
Description | Data deposited in or computed by PubChem | |
Canonical SMILES |
[B-](F)(F)(F)F.[C-]#[O+].[C-]#[O+].C1CCOC1.[CH-]1C=CC=C1.[Fe+2] | |
Source | PubChem | |
URL | https://pubchem.ncbi.nlm.nih.gov | |
Description | Data deposited in or computed by PubChem | |
Molecular Formula |
C11H13BF4FeO3 | |
Source | PubChem | |
URL | https://pubchem.ncbi.nlm.nih.gov | |
Description | Data deposited in or computed by PubChem | |
DSSTOX Substance ID |
DTXSID10746124 | |
Record name | carbon monoxide;cyclopenta-1,3-diene;iron(2+);oxolane;tetrafluoroborate | |
Source | EPA DSSTox | |
URL | https://comptox.epa.gov/dashboard/DTXSID10746124 | |
Description | DSSTox provides a high quality public chemistry resource for supporting improved predictive toxicology. | |
Molecular Weight |
335.87 g/mol | |
Source | PubChem | |
URL | https://pubchem.ncbi.nlm.nih.gov | |
Description | Data deposited in or computed by PubChem | |
CAS No. |
63313-71-3 | |
Record name | carbon monoxide;cyclopenta-1,3-diene;iron(2+);oxolane;tetrafluoroborate | |
Source | EPA DSSTox | |
URL | https://comptox.epa.gov/dashboard/DTXSID10746124 | |
Description | DSSTox provides a high quality public chemistry resource for supporting improved predictive toxicology. | |
Record name | 63313-71-3 | |
Source | European Chemicals Agency (ECHA) | |
URL | https://echa.europa.eu/information-on-chemicals | |
Description | The European Chemicals Agency (ECHA) is an agency of the European Union which is the driving force among regulatory authorities in implementing the EU's groundbreaking chemicals legislation for the benefit of human health and the environment as well as for innovation and competitiveness. | |
Explanation | Use of the information, documents and data from the ECHA website is subject to the terms and conditions of this Legal Notice, and subject to other binding limitations provided for under applicable law, the information, documents and data made available on the ECHA website may be reproduced, distributed and/or used, totally or in part, for non-commercial purposes provided that ECHA is acknowledged as the source: "Source: European Chemicals Agency, http://echa.europa.eu/". Such acknowledgement must be included in each copy of the material. ECHA permits and encourages organisations and individuals to create links to the ECHA website under the following cumulative conditions: Links can only be made to webpages that provide a link to the Legal Notice page. | |
Retrosynthesis Analysis
AI-Powered Synthesis Planning: Our tool employs the Template_relevance Pistachio, Template_relevance Bkms_metabolic, Template_relevance Pistachio_ringbreaker, Template_relevance Reaxys, Template_relevance Reaxys_biocatalysis model, leveraging a vast database of chemical reactions to predict feasible synthetic routes.
One-Step Synthesis Focus: Specifically designed for one-step synthesis, it provides concise and direct routes for your target compounds, streamlining the synthesis process.
Accurate Predictions: Utilizing the extensive PISTACHIO, BKMS_METABOLIC, PISTACHIO_RINGBREAKER, REAXYS, REAXYS_BIOCATALYSIS database, our tool offers high-accuracy predictions, reflecting the latest in chemical research and data.
Strategy Settings
Precursor scoring | Relevance Heuristic |
---|---|
Min. plausibility | 0.01 |
Model | Template_relevance |
Template Set | Pistachio/Bkms_metabolic/Pistachio_ringbreaker/Reaxys/Reaxys_biocatalysis |
Top-N result to add to graph | 6 |
Feasible Synthetic Routes
Q1: How does cyclopentadienyldicarbonyl(tetrahydrofuran)iron(II) tetrafluoroborate interact with 1,5-diaza-3,7-diphosphacyclooctanes?
A1: The research [] demonstrates that 1,5-diaza-3,7-diphosphacyclooctanes can act as ligands, replacing the tetrahydrofuran and one of the carbonyl ligands in this compound. This substitution results in the formation of new heteroligand iron complexes with the general formula [CpFeL(CO)]+BF4−, where L represents the 1,5-diaza-3,7-diphosphacyclooctane ligand.
Disclaimer and Information on In-Vitro Research Products
Please be aware that all articles and product information presented on BenchChem are intended solely for informational purposes. The products available for purchase on BenchChem are specifically designed for in-vitro studies, which are conducted outside of living organisms. In-vitro studies, derived from the Latin term "in glass," involve experiments performed in controlled laboratory settings using cells or tissues. It is important to note that these products are not categorized as medicines or drugs, and they have not received approval from the FDA for the prevention, treatment, or cure of any medical condition, ailment, or disease. We must emphasize that any form of bodily introduction of these products into humans or animals is strictly prohibited by law. It is essential to adhere to these guidelines to ensure compliance with legal and ethical standards in research and experimentation.