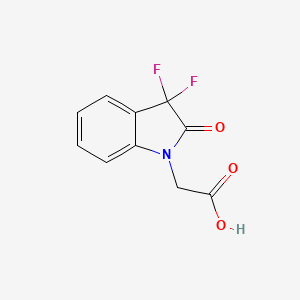
2-(3,3-Difluoro-2-oxoindolin-1-yl)acetic acid
Overview
Description
2-(3,3-Difluoro-2-oxoindolin-1-yl)acetic acid is a chemical compound characterized by its unique structure, which includes a difluoro group and an oxoindolin moiety. This compound is part of the indole derivatives family, which are known for their significant biological and pharmaceutical applications.
Mechanism of Action
Target of Action
It is known that indole derivatives, which this compound is a part of, bind with high affinity to multiple receptors . These receptors play crucial roles in various biological activities, including antiviral, anti-inflammatory, anticancer, anti-HIV, antioxidant, antimicrobial, antitubercular, antidiabetic, antimalarial, and anticholinesterase activities .
Mode of Action
Indole derivatives are known to interact with their targets, leading to changes that result in their various biological activities .
Biochemical Pathways
Indole derivatives are known to influence a variety of biochemical pathways due to their broad-spectrum biological activities .
Result of Action
Indole derivatives, including this compound, are known to have various biological activities, suggesting they have significant molecular and cellular effects .
Biochemical Analysis
Biochemical Properties
2-(3,3-Difluoro-2-oxoindolin-1-yl)acetic acid plays a significant role in various biochemical reactions. It interacts with several enzymes, proteins, and other biomolecules, influencing their activity and function. For instance, this compound has been shown to interact with enzymes involved in metabolic pathways, such as cytochrome P450 enzymes, which are responsible for the oxidation of organic substances. The nature of these interactions often involves binding to the active site of the enzyme, leading to either inhibition or activation of the enzyme’s catalytic activity .
Cellular Effects
The effects of this compound on various types of cells and cellular processes are profound. This compound can influence cell function by modulating cell signaling pathways, gene expression, and cellular metabolism. For example, it has been observed to affect the MAPK/ERK signaling pathway, which is crucial for cell proliferation and differentiation. Additionally, this compound can alter the expression of genes involved in apoptosis and cell cycle regulation, thereby impacting cell survival and growth .
Molecular Mechanism
The molecular mechanism of action of this compound involves several key processes. At the molecular level, this compound exerts its effects through binding interactions with biomolecules, enzyme inhibition or activation, and changes in gene expression. For instance, it can bind to specific receptors on the cell surface, triggering a cascade of intracellular events that lead to the activation or inhibition of downstream signaling pathways. Additionally, this compound can inhibit the activity of certain enzymes by binding to their active sites, thereby preventing substrate binding and subsequent catalysis .
Temporal Effects in Laboratory Settings
In laboratory settings, the effects of this compound can change over time. This includes its stability, degradation, and long-term effects on cellular function. Studies have shown that this compound is relatively stable under standard laboratory conditions, but it may degrade over extended periods or under specific environmental conditions, such as exposure to light or extreme pH levels. Long-term exposure to this compound has been associated with alterations in cellular metabolism and function, which can be observed in both in vitro and in vivo studies .
Dosage Effects in Animal Models
The effects of this compound vary with different dosages in animal models. At low doses, this compound may exhibit beneficial effects, such as anti-inflammatory or anticancer activities. At higher doses, it can cause toxic or adverse effects, including hepatotoxicity and nephrotoxicity. Threshold effects have been observed, where the compound’s efficacy and toxicity are dose-dependent, highlighting the importance of determining the optimal dosage for therapeutic applications .
Metabolic Pathways
This compound is involved in several metabolic pathways, interacting with various enzymes and cofactors. It can influence metabolic flux and metabolite levels by modulating the activity of key enzymes in these pathways. For example, this compound has been shown to affect the activity of enzymes involved in the tricarboxylic acid (TCA) cycle, leading to changes in the levels of intermediates and overall metabolic flux .
Transport and Distribution
The transport and distribution of this compound within cells and tissues are mediated by specific transporters and binding proteins. These interactions can affect the localization and accumulation of the compound, influencing its biological activity. For instance, the compound may be transported into cells via organic anion transporters, which facilitate its uptake and distribution within the cellular compartments .
Subcellular Localization
The subcellular localization of this compound plays a crucial role in its activity and function. This compound can be directed to specific compartments or organelles within the cell through targeting signals or post-translational modifications. For example, it may localize to the mitochondria, where it can influence mitochondrial function and energy production. The specific localization of this compound can determine its impact on cellular processes and overall cellular health .
Preparation Methods
Synthetic Routes and Reaction Conditions: The synthesis of 2-(3,3-Difluoro-2-oxoindolin-1-yl)acetic acid typically involves the following steps:
Starting Materials: The synthesis begins with the appropriate indole derivative and difluoro reagents.
Oxidation Reaction: The indole derivative undergoes an oxidation reaction to introduce the oxo group.
Substitution Reaction: The difluoro group is introduced through a substitution reaction.
Acetylation: Finally, the compound is acetylated to form the acetic acid derivative.
Industrial Production Methods: In an industrial setting, the production of this compound may involve large-scale reactions with optimized conditions to ensure high yield and purity. The use of catalysts and specific reaction conditions can enhance the efficiency of the synthesis process.
Chemical Reactions Analysis
Types of Reactions: 2-(3,3-Difluoro-2-oxoindolin-1-yl)acetic acid can undergo various chemical reactions, including:
Oxidation: The oxo group can be further oxidized to form derivatives with higher oxidation states.
Reduction: Reduction reactions can be used to modify the difluoro group.
Substitution: Substitution reactions can introduce different functional groups to the molecule.
Common Reagents and Conditions:
Oxidation: Common oxidizing agents include potassium permanganate (KMnO4) and chromium trioxide (CrO3).
Reduction: Reducing agents such as lithium aluminum hydride (LiAlH4) are often used.
Substitution: Various nucleophiles and electrophiles can be used depending on the desired substitution.
Major Products Formed: The major products formed from these reactions include various derivatives of the original compound, which can have different biological and chemical properties.
Scientific Research Applications
2-(3,3-Difluoro-2-oxoindolin-1-yl)acetic acid has several scientific research applications:
Chemistry: It is used as a building block in the synthesis of more complex molecules.
Biology: The compound can be used to study biological processes and interactions.
Industry: It can be used in the production of materials with specific properties.
Comparison with Similar Compounds
2-(3,3-Difluoro-2-oxoindolin-1-yl)acetic acid is unique due to its difluoro group and oxoindolin structure. Similar compounds include:
Indole-3-acetic acid: A naturally occurring plant hormone.
2-oxoindoline derivatives: Other derivatives of oxoindoline with different substituents.
These compounds share structural similarities but differ in their functional groups and biological activities.
Properties
IUPAC Name |
2-(3,3-difluoro-2-oxoindol-1-yl)acetic acid | |
---|---|---|
Source | PubChem | |
URL | https://pubchem.ncbi.nlm.nih.gov | |
Description | Data deposited in or computed by PubChem | |
InChI |
InChI=1S/C10H7F2NO3/c11-10(12)6-3-1-2-4-7(6)13(9(10)16)5-8(14)15/h1-4H,5H2,(H,14,15) | |
Source | PubChem | |
URL | https://pubchem.ncbi.nlm.nih.gov | |
Description | Data deposited in or computed by PubChem | |
InChI Key |
WJIHEGXZOMSHNO-UHFFFAOYSA-N | |
Source | PubChem | |
URL | https://pubchem.ncbi.nlm.nih.gov | |
Description | Data deposited in or computed by PubChem | |
Canonical SMILES |
C1=CC=C2C(=C1)C(C(=O)N2CC(=O)O)(F)F | |
Source | PubChem | |
URL | https://pubchem.ncbi.nlm.nih.gov | |
Description | Data deposited in or computed by PubChem | |
Molecular Formula |
C10H7F2NO3 | |
Source | PubChem | |
URL | https://pubchem.ncbi.nlm.nih.gov | |
Description | Data deposited in or computed by PubChem | |
Molecular Weight |
227.16 g/mol | |
Source | PubChem | |
URL | https://pubchem.ncbi.nlm.nih.gov | |
Description | Data deposited in or computed by PubChem | |
Synthesis routes and methods
Procedure details
Retrosynthesis Analysis
AI-Powered Synthesis Planning: Our tool employs the Template_relevance Pistachio, Template_relevance Bkms_metabolic, Template_relevance Pistachio_ringbreaker, Template_relevance Reaxys, Template_relevance Reaxys_biocatalysis model, leveraging a vast database of chemical reactions to predict feasible synthetic routes.
One-Step Synthesis Focus: Specifically designed for one-step synthesis, it provides concise and direct routes for your target compounds, streamlining the synthesis process.
Accurate Predictions: Utilizing the extensive PISTACHIO, BKMS_METABOLIC, PISTACHIO_RINGBREAKER, REAXYS, REAXYS_BIOCATALYSIS database, our tool offers high-accuracy predictions, reflecting the latest in chemical research and data.
Strategy Settings
Precursor scoring | Relevance Heuristic |
---|---|
Min. plausibility | 0.01 |
Model | Template_relevance |
Template Set | Pistachio/Bkms_metabolic/Pistachio_ringbreaker/Reaxys/Reaxys_biocatalysis |
Top-N result to add to graph | 6 |
Feasible Synthetic Routes
Disclaimer and Information on In-Vitro Research Products
Please be aware that all articles and product information presented on BenchChem are intended solely for informational purposes. The products available for purchase on BenchChem are specifically designed for in-vitro studies, which are conducted outside of living organisms. In-vitro studies, derived from the Latin term "in glass," involve experiments performed in controlled laboratory settings using cells or tissues. It is important to note that these products are not categorized as medicines or drugs, and they have not received approval from the FDA for the prevention, treatment, or cure of any medical condition, ailment, or disease. We must emphasize that any form of bodily introduction of these products into humans or animals is strictly prohibited by law. It is essential to adhere to these guidelines to ensure compliance with legal and ethical standards in research and experimentation.