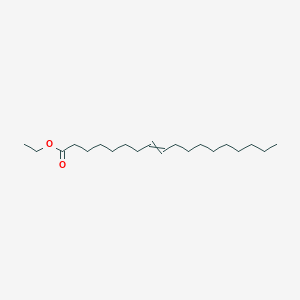
Ethyl octadec-8-enoate
- Click on QUICK INQUIRY to receive a quote from our team of experts.
- With the quality product at a COMPETITIVE price, you can focus more on your research.
Overview
Description
Ethyl octadec-8-enoate, also known as ethyl oleate, is an ester formed from the reaction of oleic acid and ethanol. It is a colorless to pale yellow liquid with a mild odor. This compound is widely used in various industries due to its unique chemical properties and versatility.
Preparation Methods
Synthetic Routes and Reaction Conditions: Ethyl octadec-8-enoate is typically synthesized through the esterification of oleic acid with ethanol. The reaction is catalyzed by an acid, such as sulfuric acid or p-toluenesulfonic acid, and is carried out under reflux conditions to drive the reaction to completion. The general reaction is as follows:
C8H17CH=CH(C7H14COOH)+C2H5OH→C8H17CH=CH(C7H14COOC2H5)+H2O
Industrial Production Methods: In industrial settings, the production of this compound often involves continuous esterification processes. These processes use large-scale reactors and efficient separation techniques to maximize yield and purity. Catalysts such as ion-exchange resins or immobilized enzymes can be employed to enhance reaction rates and selectivity.
Types of Reactions:
Oxidation: this compound can undergo oxidation reactions, particularly at the double bond, leading to the formation of epoxides or hydroxylated products.
Reduction: The double bond in this compound can be reduced to form ethyl stearate using hydrogenation catalysts like palladium on carbon.
Substitution: The ester group can participate in nucleophilic substitution reactions, where the ethoxy group is replaced by other nucleophiles.
Common Reagents and Conditions:
Oxidation: Reagents such as peracids (e.g., m-chloroperbenzoic acid) or osmium tetroxide can be used under mild conditions.
Reduction: Hydrogen gas in the presence of a palladium catalyst at elevated temperatures and pressures.
Substitution: Strong nucleophiles like alkoxides or amines under basic conditions.
Major Products:
Oxidation: Epoxides, diols, or hydroxy esters.
Reduction: Ethyl stearate.
Substitution: Various substituted esters depending on the nucleophile used.
Scientific Research Applications
Ethyl octadec-8-enoate has a wide range of applications in scientific research:
Chemistry: Used as a solvent and reagent in organic synthesis. It serves as a model compound for studying esterification and transesterification reactions.
Biology: Employed in the formulation of lipid-based drug delivery systems. It is also used in the preparation of cell culture media.
Medicine: Investigated for its potential as a biocompatible solvent in pharmaceutical formulations. It is also used in the synthesis of prodrugs.
Industry: Utilized as a plasticizer in the production of polymers and resins. It is also a component in cosmetics and personal care products due to its emollient properties.
Mechanism of Action
The mechanism by which ethyl octadec-8-enoate exerts its effects depends on its application:
In drug delivery systems: It enhances the solubility and bioavailability of hydrophobic drugs by forming stable emulsions or micelles.
In biological systems: It can integrate into lipid bilayers, affecting membrane fluidity and permeability.
In chemical reactions: It acts as a reactant or intermediate, participating in various organic transformations.
Comparison with Similar Compounds
Ethyl octadec-8-enoate can be compared with other fatty acid esters such as:
Ethyl stearate: Similar in structure but lacks the double bond, making it less reactive in certain chemical reactions.
Methyl oleate: Similar in structure but has a methyl group instead of an ethyl group, affecting its physical properties and reactivity.
Ethyl linoleate: Contains two double bonds, making it more prone to oxidation and polymerization.
Uniqueness: this compound’s unique combination of a long hydrophobic chain and a reactive double bond makes it particularly useful in applications requiring both solubility in non-polar solvents and reactivity towards various chemical transformations.
By understanding the properties, preparation methods, and applications of this compound, researchers and industry professionals can better utilize this versatile compound in their respective fields.
Properties
CAS No. |
160193-36-2 |
---|---|
Molecular Formula |
C20H38O2 |
Molecular Weight |
310.5 g/mol |
IUPAC Name |
ethyl octadec-8-enoate |
InChI |
InChI=1S/C20H38O2/c1-3-5-6-7-8-9-10-11-12-13-14-15-16-17-18-19-20(21)22-4-2/h12-13H,3-11,14-19H2,1-2H3 |
InChI Key |
VSWIUGYJLIZPHM-UHFFFAOYSA-N |
Canonical SMILES |
CCCCCCCCCC=CCCCCCCC(=O)OCC |
Origin of Product |
United States |
Disclaimer and Information on In-Vitro Research Products
Please be aware that all articles and product information presented on BenchChem are intended solely for informational purposes. The products available for purchase on BenchChem are specifically designed for in-vitro studies, which are conducted outside of living organisms. In-vitro studies, derived from the Latin term "in glass," involve experiments performed in controlled laboratory settings using cells or tissues. It is important to note that these products are not categorized as medicines or drugs, and they have not received approval from the FDA for the prevention, treatment, or cure of any medical condition, ailment, or disease. We must emphasize that any form of bodily introduction of these products into humans or animals is strictly prohibited by law. It is essential to adhere to these guidelines to ensure compliance with legal and ethical standards in research and experimentation.