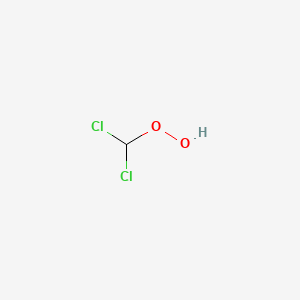
Dichloromethaneperoxol
- Click on QUICK INQUIRY to receive a quote from our team of experts.
- With the quality product at a COMPETITIVE price, you can focus more on your research.
Overview
Description
Dichloromethaneperoxol is a chemical compound that has garnered interest in various scientific fields due to its unique properties and potential applications. It is a derivative of dichloromethane, which is widely known for its use as a solvent in industrial and laboratory settings.
Preparation Methods
Synthetic Routes and Reaction Conditions
The synthesis of dichloromethaneperoxol typically involves the reaction of dichloromethane with hydrogen peroxide under controlled conditions. The reaction is carried out in the presence of a catalyst, often a transition metal complex, to facilitate the formation of the peroxol group. The reaction conditions, such as temperature and pressure, are carefully monitored to ensure the desired product is obtained with high purity.
Industrial Production Methods
In an industrial setting, the production of this compound follows a similar synthetic route but on a larger scale. The process involves the continuous feeding of dichloromethane and hydrogen peroxide into a reactor, where the reaction takes place. The product is then purified through distillation or other separation techniques to remove any impurities and unreacted starting materials.
Chemical Reactions Analysis
Types of Reactions
Dichloromethaneperoxol undergoes various chemical reactions, including:
Oxidation: It can act as an oxidizing agent, facilitating the oxidation of other compounds.
Reduction: Under certain conditions, it can be reduced to dichloromethane and water.
Substitution: It can participate in substitution reactions where the peroxol group is replaced by other functional groups.
Common Reagents and Conditions
Common reagents used in reactions with this compound include acids, bases, and other oxidizing or reducing agents. The reaction conditions, such as temperature, solvent, and pH, are optimized based on the desired reaction and product.
Major Products Formed
The major products formed from reactions involving this compound depend on the specific reaction conditions and reagents used. For example, in oxidation reactions, the major products may include oxidized organic compounds, while in reduction reactions, the products may include dichloromethane and water.
Scientific Research Applications
Dichloromethaneperoxol has a wide range of applications in scientific research, including:
Chemistry: It is used as an oxidizing agent in various organic synthesis reactions.
Biology: It is studied for its potential use in biological assays and as a reagent in biochemical reactions.
Medicine: Research is ongoing to explore its potential therapeutic applications, particularly in the field of oncology.
Industry: It is used in the production of fine chemicals and as a reagent in various industrial processes.
Mechanism of Action
The mechanism of action of dichloromethaneperoxol involves the interaction of the peroxol group with target molecules. The peroxol group can donate oxygen atoms to other molecules, facilitating oxidation reactions. This property makes it a valuable reagent in various chemical and biological processes. The molecular targets and pathways involved in its action depend on the specific application and reaction conditions.
Comparison with Similar Compounds
Similar Compounds
Compounds similar to dichloromethaneperoxol include:
Dichloromethane: A widely used solvent with similar chemical properties but lacking the peroxol group.
Hydrogen Peroxide: A common oxidizing agent that shares some reactivity with this compound.
Peracetic Acid: Another oxidizing agent with similar applications in organic synthesis.
Uniqueness
This compound is unique due to the presence of both dichloromethane and peroxol functionalities in a single molecule. This combination allows it to participate in a wider range of chemical reactions compared to its individual components. Its ability to act as both a solvent and an oxidizing agent makes it a versatile compound in various scientific and industrial applications.
Properties
CAS No. |
138434-14-7 |
---|---|
Molecular Formula |
CH2Cl2O2 |
Molecular Weight |
116.93 g/mol |
IUPAC Name |
dichloro(hydroperoxy)methane |
InChI |
InChI=1S/CH2Cl2O2/c2-1(3)5-4/h1,4H |
InChI Key |
TUIYYMKEIGYDJB-UHFFFAOYSA-N |
Canonical SMILES |
C(OO)(Cl)Cl |
Origin of Product |
United States |
Disclaimer and Information on In-Vitro Research Products
Please be aware that all articles and product information presented on BenchChem are intended solely for informational purposes. The products available for purchase on BenchChem are specifically designed for in-vitro studies, which are conducted outside of living organisms. In-vitro studies, derived from the Latin term "in glass," involve experiments performed in controlled laboratory settings using cells or tissues. It is important to note that these products are not categorized as medicines or drugs, and they have not received approval from the FDA for the prevention, treatment, or cure of any medical condition, ailment, or disease. We must emphasize that any form of bodily introduction of these products into humans or animals is strictly prohibited by law. It is essential to adhere to these guidelines to ensure compliance with legal and ethical standards in research and experimentation.