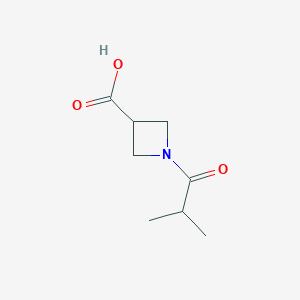
1-(2-Methylpropanoyl)azetidine-3-carboxylic acid
Overview
Description
1-(2-Methylpropanoyl)azetidine-3-carboxylic acid is a compound belonging to the azetidine family, which is characterized by a four-membered nitrogen-containing ring Azetidines are known for their significant ring strain, which imparts unique reactivity and stability properties
Mechanism of Action
Target of Action
The primary targets of azetidine derivatives, such as azetidine-2-carboxylic acid (Aze), are often proteins. Aze, for example, is an analog of the amino acid proline (Pro) and can be misincorporated during protein biosynthesis .
Mode of Action
Azetidine derivatives can inhibit the growth of organisms like plants by being misincorporated into proteins in place of proline, leading to the production of misfolded proteins .
Biochemical Pathways
Misincorporation of azetidine derivatives into proteins can affect various biochemical pathways. For instance, it can trigger the unfolded protein response, leading to protein degradation .
Result of Action
The misincorporation of azetidine derivatives into proteins can lead to the production of misfolded proteins, which can disrupt cellular functions and inhibit growth .
Action Environment
The action, efficacy, and stability of azetidine derivatives can be influenced by various environmental factors. For instance, the presence of proline can restore growth reduction caused by Aze .
Biochemical Analysis
Biochemical Properties
1-(2-Methylpropanoyl)azetidine-3-carboxylic acid plays a crucial role in biochemical reactions due to its ability to interact with a variety of enzymes, proteins, and other biomolecules. One of the key interactions involves its incorporation into proteins in place of proline, a process facilitated by its structural similarity to proline. This misincorporation can lead to protein misfolding and altered protein function. Additionally, this compound has been shown to interact with enzymes involved in amino acid metabolism, such as proline dehydrogenase, affecting the catalytic activity and stability of these enzymes .
Cellular Effects
The effects of this compound on various cell types and cellular processes are profound. In mammalian cells, this compound can induce endoplasmic reticulum (ER) stress by promoting the accumulation of misfolded proteins. This stress response activates the unfolded protein response (UPR), leading to changes in gene expression and cellular metabolism. Additionally, this compound has been observed to trigger pro-inflammatory and pro-apoptotic pathways in microglial cells, resulting in increased expression of inflammatory cytokines and apoptosis markers .
Molecular Mechanism
At the molecular level, this compound exerts its effects through several mechanisms. Its structural mimicry of proline allows it to be incorporated into proteins, disrupting normal protein folding and function. This misincorporation can inhibit or activate various enzymes, depending on the context. For example, it can inhibit proline dehydrogenase by competing with proline for binding sites, leading to reduced enzyme activity. Additionally, the compound can induce changes in gene expression by activating stress response pathways, such as the UPR, which modulates the expression of genes involved in protein folding, degradation, and apoptosis .
Temporal Effects in Laboratory Settings
The stability and effects of this compound over time have been studied in various laboratory settings. This compound is relatively stable under physiological conditions but can undergo degradation under extreme pH or temperature conditions. Long-term exposure to this compound in cell culture models has shown sustained activation of stress response pathways and persistent changes in cellular function, including altered metabolism and increased apoptosis .
Dosage Effects in Animal Models
The effects of this compound vary with dosage in animal models. At low doses, the compound can induce mild stress responses and minor changes in cellular function. At higher doses, it can cause significant toxicity, including severe ER stress, widespread apoptosis, and organ damage. Studies in mice have shown that high doses of this compound can lead to neurological deficits and impaired motor function, highlighting the importance of dosage in determining the compound’s effects .
Metabolic Pathways
This compound is involved in several metabolic pathways, primarily those related to amino acid metabolism. It can be metabolized by enzymes such as proline dehydrogenase and pyrroline-5-carboxylate reductase, leading to the formation of various metabolites. These metabolic processes can affect the levels of other metabolites and influence metabolic flux within the cell. Additionally, the compound’s interaction with metabolic enzymes can alter their activity and stability, further impacting cellular metabolism .
Transport and Distribution
The transport and distribution of this compound within cells and tissues are mediated by specific transporters and binding proteins. This compound can be taken up by cells via amino acid transporters, such as the proline transporter, and distributed to various cellular compartments. Its localization within the cell can influence its activity and interactions with other biomolecules. For example, its accumulation in the ER can exacerbate ER stress and promote the activation of stress response pathways .
Subcellular Localization
The subcellular localization of this compound is primarily within the endoplasmic reticulum, where it can exert its effects on protein folding and stress response pathways. The compound’s localization is influenced by targeting signals and post-translational modifications that direct it to specific cellular compartments. Its presence in the ER can lead to the activation of the UPR and other stress response pathways, ultimately affecting cellular function and viability .
Preparation Methods
Synthetic Routes and Reaction Conditions: The synthesis of 1-(2-Methylpropanoyl)azetidine-3-carboxylic acid typically involves the formation of the azetidine ring followed by functionalization at the 3-position. One common method involves the [2+2] cycloaddition of imines with alkenes to form the azetidine ring. Subsequent acylation and carboxylation reactions introduce the 2-methylpropanoyl and carboxylic acid groups, respectively .
Industrial Production Methods: Industrial production of this compound may involve optimized versions of the laboratory synthesis methods, with a focus on scalability, cost-effectiveness, and environmental considerations. Catalysts and solvents are chosen to maximize yield and minimize waste, and continuous flow reactors may be employed to enhance efficiency .
Chemical Reactions Analysis
Types of Reactions: 1-(2-Methylpropanoyl)azetidine-3-carboxylic acid undergoes various types of chemical reactions, including:
Oxidation: The compound can be oxidized to introduce additional functional groups or modify existing ones.
Reduction: Reduction reactions can be used to alter the oxidation state of the compound, potentially leading to different reactivity profiles.
Substitution: Nucleophilic and electrophilic substitution reactions can occur at various positions on the azetidine ring
Common Reagents and Conditions:
Oxidation: Common oxidizing agents include potassium permanganate (KMnO4) and chromium trioxide (CrO3).
Reduction: Reducing agents such as lithium aluminum hydride (LiAlH4) and sodium borohydride (NaBH4) are frequently used.
Substitution: Reagents like halogens (e.g., chlorine, bromine) and nucleophiles (e.g., amines, alcohols) are commonly employed
Major Products: The major products formed from these reactions depend on the specific conditions and reagents used. For example, oxidation may yield carboxylic acids or ketones, while reduction could produce alcohols or amines .
Scientific Research Applications
1-(2-Methylpropanoyl)azetidine-3-carboxylic acid has a wide range of scientific research applications:
Chemistry: It serves as a building block for the synthesis of more complex molecules, including pharmaceuticals and agrochemicals.
Biology: The compound’s unique structure allows it to interact with biological molecules in specific ways, making it useful in the study of enzyme mechanisms and protein-ligand interactions.
Industry: It can be used in the production of polymers and other materials with specialized properties
Comparison with Similar Compounds
Azetidine-2-carboxylic acid: Another azetidine derivative, known for its role as a proline analog in biological systems.
1-(2-Methylpropanoyl)azetidine: Similar to the compound but lacks the carboxylic acid group.
Azetidine-3-carboxylic acid: Similar structure but without the 2-methylpropanoyl group
Uniqueness: 1-(2-Methylpropanoyl)azetidine-3-carboxylic acid is unique due to the presence of both the 2-methylpropanoyl and carboxylic acid groups, which confer distinct chemical and biological properties. These functional groups enhance the compound’s reactivity and potential for interaction with biological targets, making it a valuable tool in various research and industrial applications .
Properties
IUPAC Name |
1-(2-methylpropanoyl)azetidine-3-carboxylic acid | |
---|---|---|
Source | PubChem | |
URL | https://pubchem.ncbi.nlm.nih.gov | |
Description | Data deposited in or computed by PubChem | |
InChI |
InChI=1S/C8H13NO3/c1-5(2)7(10)9-3-6(4-9)8(11)12/h5-6H,3-4H2,1-2H3,(H,11,12) | |
Source | PubChem | |
URL | https://pubchem.ncbi.nlm.nih.gov | |
Description | Data deposited in or computed by PubChem | |
InChI Key |
HLGCTGZBJCWEFL-UHFFFAOYSA-N | |
Source | PubChem | |
URL | https://pubchem.ncbi.nlm.nih.gov | |
Description | Data deposited in or computed by PubChem | |
Canonical SMILES |
CC(C)C(=O)N1CC(C1)C(=O)O | |
Source | PubChem | |
URL | https://pubchem.ncbi.nlm.nih.gov | |
Description | Data deposited in or computed by PubChem | |
Molecular Formula |
C8H13NO3 | |
Source | PubChem | |
URL | https://pubchem.ncbi.nlm.nih.gov | |
Description | Data deposited in or computed by PubChem | |
Molecular Weight |
171.19 g/mol | |
Source | PubChem | |
URL | https://pubchem.ncbi.nlm.nih.gov | |
Description | Data deposited in or computed by PubChem | |
Retrosynthesis Analysis
AI-Powered Synthesis Planning: Our tool employs the Template_relevance Pistachio, Template_relevance Bkms_metabolic, Template_relevance Pistachio_ringbreaker, Template_relevance Reaxys, Template_relevance Reaxys_biocatalysis model, leveraging a vast database of chemical reactions to predict feasible synthetic routes.
One-Step Synthesis Focus: Specifically designed for one-step synthesis, it provides concise and direct routes for your target compounds, streamlining the synthesis process.
Accurate Predictions: Utilizing the extensive PISTACHIO, BKMS_METABOLIC, PISTACHIO_RINGBREAKER, REAXYS, REAXYS_BIOCATALYSIS database, our tool offers high-accuracy predictions, reflecting the latest in chemical research and data.
Strategy Settings
Precursor scoring | Relevance Heuristic |
---|---|
Min. plausibility | 0.01 |
Model | Template_relevance |
Template Set | Pistachio/Bkms_metabolic/Pistachio_ringbreaker/Reaxys/Reaxys_biocatalysis |
Top-N result to add to graph | 6 |
Feasible Synthetic Routes
Disclaimer and Information on In-Vitro Research Products
Please be aware that all articles and product information presented on BenchChem are intended solely for informational purposes. The products available for purchase on BenchChem are specifically designed for in-vitro studies, which are conducted outside of living organisms. In-vitro studies, derived from the Latin term "in glass," involve experiments performed in controlled laboratory settings using cells or tissues. It is important to note that these products are not categorized as medicines or drugs, and they have not received approval from the FDA for the prevention, treatment, or cure of any medical condition, ailment, or disease. We must emphasize that any form of bodily introduction of these products into humans or animals is strictly prohibited by law. It is essential to adhere to these guidelines to ensure compliance with legal and ethical standards in research and experimentation.