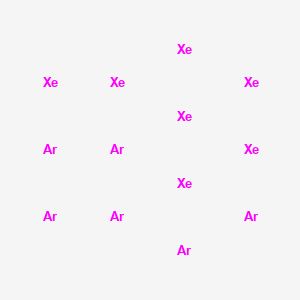
Argon;xenon
- Click on QUICK INQUIRY to receive a quote from our team of experts.
- With the quality product at a COMPETITIVE price, you can focus more on your research.
Overview
Description
Argon (Ar) and xenon (Xe) are noble gases in Group 18 of the periodic table. Both are monatomic, colorless, odorless, and chemically inert under standard conditions.
- Argon: Discovered in 1894, argon is the third-most abundant gas in Earth’s atmosphere (≈0.934%). It has an atomic number of 18, atomic weight of 39.948 g/mol, and a boiling point of -185.8°C. Applications range from industrial welding to medical neuroprotection research .
- Xenon: First isolated in 1898, xenon is rare in Earth’s atmosphere (≈0.087 ppm). It has an atomic number of 54, atomic weight of 131.29 g/mol, and a boiling point of -108.1°C. Xenon is used in lighting, medical imaging, and anesthesia due to its unique physicochemical properties .
Preparation Methods
Synthetic Routes and Reaction Conditions: The synthesis of Argon-xenon compounds typically involves high-pressure conditions. Using unbiased structure searching techniques combined with first-principles calculations, it has been demonstrated that argon can react with xenon at pressures as low as 1.1 GPa, producing a novel van der Waals compound XeAr₂ . This compound crystallizes in the MgCu₂-type Laves phase structure and remains stable without any phase transition or decomposition up to 500 GPa .
Industrial Production Methods: Industrial production of Argon-xenon compounds is not common due to the rarity and high cost of xenon. the separation and purification of xenon from noble gas mixtures, including argon, can be achieved using gas hydrate technology. This method presents a 20% energy cost advantage over traditional cryogenic distillation .
Chemical Reactions Analysis
Types of Reactions: Argon-xenon compounds primarily undergo van der Waals interactions rather than traditional chemical reactions such as oxidation, reduction, or substitution. The compound XeAr₂ is a wide-gap insulator and does not exhibit significant reactivity under normal conditions .
Common Reagents and Conditions: The formation of Argon-xenon compounds requires high-pressure conditions. No specific reagents are involved in the synthesis, as the reaction relies on the physical interaction between argon and xenon atoms under pressure .
Major Products Formed: The primary product formed from the reaction of argon and xenon is the van der Waals compound XeAr₂. This compound is stable and does not decompose or undergo phase transitions up to 500 GPa .
Scientific Research Applications
Argon-xenon compounds have potential applications in various scientific fields. In chemistry, they provide insights into the behavior of noble gases under high-pressure conditions and contribute to the understanding of van der Waals interactions. In the medical field, xenon is used as an anesthetic and in imaging techniques, while argon is used in cryogenics and laser technology . The unique properties of Argon-xenon compounds may lead to new applications in these areas.
Mechanism of Action
The mechanism of action of Argon-xenon compounds involves van der Waals interactions between the argon and xenon atoms. Under high pressure, the electrons in the outermost shells of these atoms become delocalized, leading to the formation of covalent Xe-Xe and Xe-Ar bonds . This delocalization stabilizes the compound and prevents decomposition or phase transitions up to 500 GPa .
Comparison with Similar Compounds
Comparison of Physical Properties
Atomic and Thermophysical Characteristics
Xenon’s larger atomic radius and higher polarizability result in stronger van der Waals interactions, explaining its higher boiling point and distinct adsorption behavior compared to argon .
Behavior Under Extreme Conditions
In radiative shock simulations, xenon exhibits a higher peak electron temperature (23.9–25.8 eV vs. 18.3–22.1 eV for argon) and ionization state (Xe⁺⁹ vs. Ar⁺⁶). Xenon also produces a larger precursor region due to enhanced radiative losses .
Chemical Reactivity and Compound Formation
Stability of Noble Gas Compounds
- Xenon: Forms stable compounds under high-pressure conditions (e.g., XeFe₃ and XeNi₃ in Earth’s core) and reacts with electronegative elements like fluorine and oxygen (e.g., XeO₃, XeF₄) .
- Argon: No stable bulk compounds are known under ambient conditions. Computational studies confirm argon’s inability to form analogous compounds to xenon, even at extreme pressures .
Neuroprotective Effects in Medical Research
Efficacy in Preclinical Models
A meta-analysis of 32 studies comparing argon and xenon in neuroprotection revealed:
- Xenon: Effect size = 34.1% (95% CI: 24.7–43.6%) in cardiac arrest (CA), traumatic brain injury (TBI), and stroke models.
- Argon: Effect size = 18.1% (95% CI: 8.1–28.1%), 1.9-fold lower than xenon (P < 0.001) .
Clinical Translation Challenges
Despite xenon’s superior efficacy, its high cost has driven research into argon as a cheaper alternative. However, argon’s effect size (15–25% reduction in brain cell death) remains significantly lower .
Gas Purity and Separation
Fractional distillation reduces xenon impurities in argon from 140 ppb to 7 ppb, critical for high-purity applications like semiconductor manufacturing .
Adsorption on Zeolites
Xenon requires a steric factor of 0.76 for adsorption on 5A zeolites, while argon and krypton exhibit near-ideal adsorption without such adjustments .
Q & A
Basic Research Questions
Q. What are the standard methodologies for isolating and purifying argon and xenon in laboratory settings?
- Methodological Answer : Cryogenic distillation is widely used due to the distinct boiling points of noble gases (Ar: −185.8°C; Xe: −108.1°C). For ultra-high-purity requirements, gas chromatography coupled with gettering (e.g., titanium sublimation pumps) removes trace contaminants like oxygen or nitrogen . Validation of purity should involve mass spectrometry or residual gas analysis, with calibration against certified reference materials .
Q. How do researchers control variables when studying argon-xenon mixtures in gas-filled detectors?
- Methodological Answer : Key variables include gas pressure, temperature, and mixing ratios. Use mass flow controllers (±1% accuracy) for precise gas blending. Stabilize environmental conditions via temperature-controlled chambers (±0.1°C). Document deviations using error propagation models (e.g., Monte Carlo simulations) .
Q. What statistical approaches are recommended for analyzing ionization efficiency data in argon-xenon plasma studies?
- Methodological Answer : Apply ANOVA to compare ionization yields across multiple voltage gradients or gas ratios. For non-normal distributions, non-parametric tests (e.g., Kruskal-Wallis) are preferable. Report confidence intervals (95%) and effect sizes to contextualize practical significance .
Advanced Research Questions
Q. How can conflicting data on xenon’s diffusion coefficients in argon matrices be resolved?
- Methodological Answer : Conduct a systematic meta-analysis of literature data, categorizing results by experimental setups (e.g., time-of-flight vs. tracer methods). Replicate experiments under identical conditions (pressure, temperature) while using traceable calibration standards. Discrepancies may arise from impurities; thus, include gas purity certifications (≥99.999%) in reporting .
Q. What experimental designs mitigate argon’s quenching effects in xenon-based scintillation detectors?
- Methodological Answer : Optimize gas ratios using a factorial design (e.g., 90% Xe/10% Ar vs. 95% Xe/5% Ar). Measure light yield and temporal resolution via photomultiplier tubes (PMTs) with nanosecond timing precision. Compare results against Monte Carlo simulations (e.g., Geant4) to isolate quenching contributions .
Q. How should researchers validate computational models of argon-xenon phase diagrams under extreme pressures?
- Methodological Answer : Use diamond anvil cells (DACs) to replicate high-pressure conditions (up to 100 GPa). Validate models (e.g., density functional theory) against synchrotron X-ray diffraction data. Discrepancies in predicted vs. observed phase boundaries require re-evaluating intermolecular potential parameters .
Q. Data Contradiction and Synthesis
Q. What protocols address inconsistencies in reported argon-xenon reaction cross-sections with metastable species?
- Methodological Answer : Cross-validate data using independent techniques (e.g., beam-gas experiments vs. laser-induced fluorescence). Assess systematic errors via inter-laboratory comparisons. Publish raw datasets with metadata (e.g., beam energy calibration logs) to enable reproducibility .
Q. How can literature reviews systematically integrate heterogeneous studies on argon-xenon excimer formation?
- Methodological Answer : Adopt PRISMA guidelines for systematic reviews. Use keyword strategies (e.g., "argon-xenon excimer" AND "ultraviolet emission") across Scopus, Web of Science, and INIS. Categorize findings by excitation methods (e.g., pulsed discharges, electron beams) and critically appraise experimental rigor using tools like ROBIS .
Q. Experimental Design and Validation
Q. What steps ensure reproducibility in cryogenic systems for argon-xenon mixture studies?
- Methodological Answer : Document thermal anchoring strategies to minimize temperature gradients. Use cryogenic pressure sensors with ±0.1 kPa accuracy. Include a detailed supplementary section on cryostat calibration procedures and vacuum integrity tests (e.g., helium leak detection) .
Q. How should researchers design hypotheses for argon-xenon interactions in astrophysical analog experiments?
- Methodological Answer : Frame hypotheses around specific astrophysical conditions (e.g., interstellar medium vs. planetary atmospheres). Use scaling laws to simulate low-density environments (≤10⁻⁶ Torr). Validate via spectroscopic comparisons with observational data (e.g., JWST archival spectra) .
Q. Tables: Key Methodological Considerations
Properties
CAS No. |
220152-42-1 |
---|---|
Molecular Formula |
Ar6Xe7 |
Molecular Weight |
1158 g/mol |
IUPAC Name |
argon;xenon |
InChI |
InChI=1S/6Ar.7Xe |
InChI Key |
FONSNBRKNKYKTR-UHFFFAOYSA-N |
Canonical SMILES |
[Ar].[Ar].[Ar].[Ar].[Ar].[Ar].[Xe].[Xe].[Xe].[Xe].[Xe].[Xe].[Xe] |
Origin of Product |
United States |
Disclaimer and Information on In-Vitro Research Products
Please be aware that all articles and product information presented on BenchChem are intended solely for informational purposes. The products available for purchase on BenchChem are specifically designed for in-vitro studies, which are conducted outside of living organisms. In-vitro studies, derived from the Latin term "in glass," involve experiments performed in controlled laboratory settings using cells or tissues. It is important to note that these products are not categorized as medicines or drugs, and they have not received approval from the FDA for the prevention, treatment, or cure of any medical condition, ailment, or disease. We must emphasize that any form of bodily introduction of these products into humans or animals is strictly prohibited by law. It is essential to adhere to these guidelines to ensure compliance with legal and ethical standards in research and experimentation.