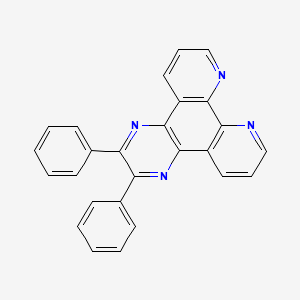
2,3-Diphenyl-1,4,8,9-tetraazatriphenylene
- Click on QUICK INQUIRY to receive a quote from our team of experts.
- With the quality product at a COMPETITIVE price, you can focus more on your research.
Overview
Description
2,3-Diphenyl-1,4,8,9-tetraazatriphenylene is a polycyclic aromatic compound known for its rigid, planar structure and excellent π–π stacking ability. This compound is part of the hexaazatriphenylene (HAT) family, which is characterized by its electron-deficient nature and aromatic discotic system .
Preparation Methods
Synthetic Routes and Reaction Conditions
The synthesis of 2,3-Diphenyl-1,4,8,9-tetraazatriphenylene typically involves the cyclization of appropriate precursors under specific conditions. One common method includes the use of dipyrazinoquinoxaline derivatives, which undergo cyclization in the presence of a suitable catalyst . The reaction conditions often involve high temperatures and inert atmospheres to ensure the stability of the intermediates and the final product.
Industrial Production Methods
Industrial production methods for this compound are not widely documented, but they likely involve scalable versions of the laboratory synthesis methods. These methods would need to be optimized for yield, purity, and cost-effectiveness, potentially involving continuous flow reactors and automated systems to handle the complex reaction conditions.
Chemical Reactions Analysis
Types of Reactions
2,3-Diphenyl-1,4,8,9-tetraazatriphenylene can undergo various chemical reactions, including:
Oxidation: This reaction can introduce oxygen-containing functional groups into the molecule.
Reduction: This reaction can remove oxygen-containing functional groups or reduce double bonds.
Substitution: This reaction can replace hydrogen atoms with other functional groups, such as halogens or alkyl groups.
Common Reagents and Conditions
Common reagents used in these reactions include oxidizing agents like potassium permanganate for oxidation, reducing agents like lithium aluminum hydride for reduction, and halogenating agents like bromine for substitution reactions. The conditions typically involve controlled temperatures and inert atmospheres to prevent unwanted side reactions.
Major Products
The major products formed from these reactions depend on the specific reagents and conditions used. For example, oxidation can yield quinoxaline derivatives, while substitution reactions can produce halogenated or alkylated derivatives .
Scientific Research Applications
2,3-Diphenyl-1,4,8,9-tetraazatriphenylene has a wide range of scientific research applications:
Mechanism of Action
The mechanism by which 2,3-Diphenyl-1,4,8,9-tetraazatriphenylene exerts its effects is primarily through its ability to engage in π–π stacking interactions. This allows it to interact with various molecular targets, including DNA and proteins, potentially stabilizing certain structures or inhibiting specific pathways . The exact molecular targets and pathways involved can vary depending on the specific application and derivative used.
Comparison with Similar Compounds
Similar Compounds
1,4,5,8,9,12-Hexaazatriphenylene (HAT): Similar in structure but with additional nitrogen atoms, making it more electron-deficient.
Tetraphenylene: Another polycyclic aromatic compound with a different ring structure and properties.
Uniqueness
2,3-Diphenyl-1,4,8,9-tetraazatriphenylene is unique due to its specific arrangement of nitrogen atoms and phenyl groups, which confer distinct electronic properties and reactivity. Its ability to form stable π–π interactions makes it particularly valuable in materials science and molecular design .
Biological Activity
2,3-Diphenyl-1,4,8,9-tetraazatriphenylene (DPTATP) is a polycyclic aromatic compound that has garnered attention for its potential biological activities. This article delves into its biological properties, including DNA binding affinity, potential as a photosensitizer in photodynamic therapy (PDT), and other pharmacological effects.
Chemical Structure and Properties
DPTATP is characterized by its tetraazatriphenylene structure, which consists of a triphenylene core with four nitrogen atoms incorporated into the ring system. This unique structure contributes to its electronic properties and biological interactions.
1. DNA Binding Affinity
Research indicates that DPTATP exhibits significant DNA binding capabilities. It has been shown to intercalate within the DNA structure, which can lead to alterations in DNA conformation and function. A study demonstrated that DPTATP binds to calf thymus DNA with a higher affinity compared to other related compounds, suggesting its potential use in targeting DNA for therapeutic purposes .
2. Photodynamic Therapy (PDT)
DPTATP has been explored as a photosensitizer in PDT due to its ability to generate reactive oxygen species (ROS) upon light activation. The efficacy of DPTATP in PDT is quantified using the Photodynamic Threshold Model, which assesses the light dose required to achieve cell death. Preliminary data indicate that DPTATP requires lower light doses compared to traditional photosensitizers, making it a promising candidate for cancer treatment .
3. Anticancer Potential
In vitro studies have shown that DPTATP exhibits cytotoxic effects on various cancer cell lines. The compound's mechanism of action appears to involve both direct cytotoxicity and the induction of apoptosis through ROS generation. Table 1 summarizes the cytotoxic effects observed across different cell lines.
Cell Line | IC50 (µM) | Mechanism of Action |
---|---|---|
A549 | 5.2 | ROS generation and apoptosis |
HeLa | 3.8 | Intercalation and DNA damage |
MCF-7 | 6.5 | Induction of oxidative stress |
Case Studies
Several case studies have highlighted the therapeutic potential of DPTATP:
- Case Study 1 : In a study involving A549 lung cancer cells, treatment with DPTATP resulted in a significant reduction in cell viability after exposure to light, demonstrating its effectiveness as a PDT agent .
- Case Study 2 : Another investigation focused on HeLa cells revealed that DPTATP not only inhibited cell proliferation but also induced apoptosis through ROS-mediated pathways .
Properties
CAS No. |
251985-00-9 |
---|---|
Molecular Formula |
C26H16N4 |
Molecular Weight |
384.4 g/mol |
IUPAC Name |
2,3-diphenylpyrazino[2,3-f][1,10]phenanthroline |
InChI |
InChI=1S/C26H16N4/c1-3-9-17(10-4-1)21-22(18-11-5-2-6-12-18)30-26-20-14-8-16-28-24(20)23-19(25(26)29-21)13-7-15-27-23/h1-16H |
InChI Key |
ABDGYSWVSZKFTF-UHFFFAOYSA-N |
Canonical SMILES |
C1=CC=C(C=C1)C2=NC3=C(C4=C(C5=C3C=CC=N5)N=CC=C4)N=C2C6=CC=CC=C6 |
Origin of Product |
United States |
Disclaimer and Information on In-Vitro Research Products
Please be aware that all articles and product information presented on BenchChem are intended solely for informational purposes. The products available for purchase on BenchChem are specifically designed for in-vitro studies, which are conducted outside of living organisms. In-vitro studies, derived from the Latin term "in glass," involve experiments performed in controlled laboratory settings using cells or tissues. It is important to note that these products are not categorized as medicines or drugs, and they have not received approval from the FDA for the prevention, treatment, or cure of any medical condition, ailment, or disease. We must emphasize that any form of bodily introduction of these products into humans or animals is strictly prohibited by law. It is essential to adhere to these guidelines to ensure compliance with legal and ethical standards in research and experimentation.