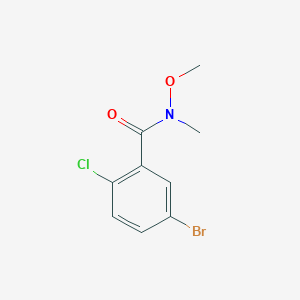
5-Bromo-2-chloro-N-methoxy-N-methylbenzamide
Overview
Description
5-Bromo-2-chloro-N-methoxy-N-methylbenzamide is an organic compound with the molecular formula C9H9BrClNO It is a derivative of benzamide, featuring bromine and chlorine substituents on the benzene ring, along with methoxy and methyl groups attached to the amide nitrogen
Preparation Methods
Synthetic Routes and Reaction Conditions
The synthesis of 5-Bromo-2-chloro-N-methoxy-N-methylbenzamide typically involves the following steps:
Starting Material: The synthesis begins with 5-bromo-2-chlorobenzoic acid.
Amidation: The carboxylic acid group is converted to an amide using N-methoxy-N-methylamine in the presence of a coupling agent such as EDCI (1-ethyl-3-(3-dimethylaminopropyl)carbodiimide) and a base like triethylamine.
Purification: The product is purified using recrystallization or chromatography techniques to obtain the desired compound with high purity.
Industrial Production Methods
Industrial production methods for this compound may involve large-scale synthesis using similar reaction conditions as described above, but optimized for higher yields and efficiency. This includes the use of automated reactors, continuous flow systems, and advanced purification techniques to ensure consistent quality and scalability.
Chemical Reactions Analysis
Types of Reactions
5-Bromo-2-chloro-N-methoxy-N-methylbenzamide can undergo various chemical reactions, including:
Substitution Reactions: The bromine and chlorine atoms on the benzene ring can be substituted with other nucleophiles under appropriate conditions.
Oxidation and Reduction: The compound can participate in oxidation and reduction reactions, altering the oxidation state of the substituents.
Hydrolysis: The amide bond can be hydrolyzed under acidic or basic conditions to yield the corresponding carboxylic acid and amine.
Common Reagents and Conditions
Nucleophilic Substitution: Reagents such as sodium methoxide or potassium tert-butoxide can be used for substitution reactions.
Oxidation: Oxidizing agents like potassium permanganate or chromium trioxide can be employed.
Reduction: Reducing agents such as lithium aluminum hydride or sodium borohydride are commonly used.
Hydrolysis: Acidic conditions (e.g., hydrochloric acid) or basic conditions (e.g., sodium hydroxide) can facilitate hydrolysis.
Major Products
Substitution: Products depend on the nucleophile used, resulting in various substituted benzamides.
Oxidation: Oxidized products may include benzoic acid derivatives.
Reduction: Reduced products may include amine derivatives.
Hydrolysis: Hydrolysis yields 5-bromo-2-chlorobenzoic acid and N-methoxy-N-methylamine.
Scientific Research Applications
5-Bromo-2-chloro-N-methoxy-N-methylbenzamide has several applications in scientific research:
Chemistry: Used as a building block in organic synthesis for the preparation of more complex molecules.
Biology: Investigated for its potential biological activity and interactions with biomolecules.
Medicine: Explored for its potential therapeutic properties, including as a precursor for drug development.
Industry: Utilized in the synthesis of specialty chemicals and materials.
Mechanism of Action
The mechanism of action of 5-Bromo-2-chloro-N-methoxy-N-methylbenzamide involves its interaction with specific molecular targets, such as enzymes or receptors. The exact pathways and targets depend on the context of its use, whether in biological systems or chemical reactions. Detailed studies are required to elucidate the precise mechanisms and effects.
Comparison with Similar Compounds
Similar Compounds
5-Bromo-2-chloro-N,N-dimethylbenzamide: Similar structure but with two methyl groups on the amide nitrogen.
5-Bromo-2-chloro-N-methylbenzamide: Lacks the methoxy group, making it less sterically hindered.
Uniqueness
5-Bromo-2-chloro-N-methoxy-N-methylbenzamide is unique due to the presence of both methoxy and methyl groups on the amide nitrogen, which can influence its reactivity and interactions compared to other similar compounds. This structural uniqueness can lead to different chemical and biological properties, making it a valuable compound for research and development.
Properties
IUPAC Name |
5-bromo-2-chloro-N-methoxy-N-methylbenzamide | |
---|---|---|
Source | PubChem | |
URL | https://pubchem.ncbi.nlm.nih.gov | |
Description | Data deposited in or computed by PubChem | |
InChI |
InChI=1S/C9H9BrClNO2/c1-12(14-2)9(13)7-5-6(10)3-4-8(7)11/h3-5H,1-2H3 | |
Source | PubChem | |
URL | https://pubchem.ncbi.nlm.nih.gov | |
Description | Data deposited in or computed by PubChem | |
InChI Key |
VUXHGIZYRNNOSE-UHFFFAOYSA-N | |
Source | PubChem | |
URL | https://pubchem.ncbi.nlm.nih.gov | |
Description | Data deposited in or computed by PubChem | |
Canonical SMILES |
CN(C(=O)C1=C(C=CC(=C1)Br)Cl)OC | |
Source | PubChem | |
URL | https://pubchem.ncbi.nlm.nih.gov | |
Description | Data deposited in or computed by PubChem | |
Molecular Formula |
C9H9BrClNO2 | |
Source | PubChem | |
URL | https://pubchem.ncbi.nlm.nih.gov | |
Description | Data deposited in or computed by PubChem | |
DSSTOX Substance ID |
DTXSID40697413 | |
Record name | 5-Bromo-2-chloro-N-methoxy-N-methylbenzamide | |
Source | EPA DSSTox | |
URL | https://comptox.epa.gov/dashboard/DTXSID40697413 | |
Description | DSSTox provides a high quality public chemistry resource for supporting improved predictive toxicology. | |
Molecular Weight |
278.53 g/mol | |
Source | PubChem | |
URL | https://pubchem.ncbi.nlm.nih.gov | |
Description | Data deposited in or computed by PubChem | |
CAS No. |
842136-59-8 | |
Record name | 5-Bromo-2-chloro-N-methoxy-N-methylbenzamide | |
Source | CAS Common Chemistry | |
URL | https://commonchemistry.cas.org/detail?cas_rn=842136-59-8 | |
Description | CAS Common Chemistry is an open community resource for accessing chemical information. Nearly 500,000 chemical substances from CAS REGISTRY cover areas of community interest, including common and frequently regulated chemicals, and those relevant to high school and undergraduate chemistry classes. This chemical information, curated by our expert scientists, is provided in alignment with our mission as a division of the American Chemical Society. | |
Explanation | The data from CAS Common Chemistry is provided under a CC-BY-NC 4.0 license, unless otherwise stated. | |
Record name | 5-Bromo-2-chloro-N-methoxy-N-methylbenzamide | |
Source | EPA DSSTox | |
URL | https://comptox.epa.gov/dashboard/DTXSID40697413 | |
Description | DSSTox provides a high quality public chemistry resource for supporting improved predictive toxicology. | |
Synthesis routes and methods I
Procedure details
Synthesis routes and methods II
Procedure details
Retrosynthesis Analysis
AI-Powered Synthesis Planning: Our tool employs the Template_relevance Pistachio, Template_relevance Bkms_metabolic, Template_relevance Pistachio_ringbreaker, Template_relevance Reaxys, Template_relevance Reaxys_biocatalysis model, leveraging a vast database of chemical reactions to predict feasible synthetic routes.
One-Step Synthesis Focus: Specifically designed for one-step synthesis, it provides concise and direct routes for your target compounds, streamlining the synthesis process.
Accurate Predictions: Utilizing the extensive PISTACHIO, BKMS_METABOLIC, PISTACHIO_RINGBREAKER, REAXYS, REAXYS_BIOCATALYSIS database, our tool offers high-accuracy predictions, reflecting the latest in chemical research and data.
Strategy Settings
Precursor scoring | Relevance Heuristic |
---|---|
Min. plausibility | 0.01 |
Model | Template_relevance |
Template Set | Pistachio/Bkms_metabolic/Pistachio_ringbreaker/Reaxys/Reaxys_biocatalysis |
Top-N result to add to graph | 6 |
Feasible Synthetic Routes
Disclaimer and Information on In-Vitro Research Products
Please be aware that all articles and product information presented on BenchChem are intended solely for informational purposes. The products available for purchase on BenchChem are specifically designed for in-vitro studies, which are conducted outside of living organisms. In-vitro studies, derived from the Latin term "in glass," involve experiments performed in controlled laboratory settings using cells or tissues. It is important to note that these products are not categorized as medicines or drugs, and they have not received approval from the FDA for the prevention, treatment, or cure of any medical condition, ailment, or disease. We must emphasize that any form of bodily introduction of these products into humans or animals is strictly prohibited by law. It is essential to adhere to these guidelines to ensure compliance with legal and ethical standards in research and experimentation.