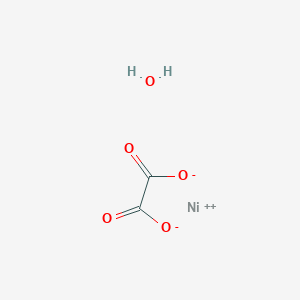
Nickel(II) oxalate hydrate
Overview
Description
Nickel(II) oxalate hydrate is a compound with the molecular formula C2NiO4 . It is also known as nickel (2+) oxalate . It is a green crystalline powder and is highly insoluble in water .
Synthesis Analysis
Nickel(II) oxalate hydrate can be synthesized from an extract solution of nickel laterite using the precipitation method . The best conditions for nickel oxalate synthesis have been determined through experimentation .Molecular Structure Analysis
Anhydrous nickel oxalate has a monoclinic crystal structure (P2 1/c, sg 14) . The carbon–carbon bond is the weakest one .Chemical Reactions Analysis
Nickel(II) oxalate hydrate can participate in various chemical reactions. For instance, it can be used as an efficient non-precious electrocatalyst for an improved alkaline oxygen evolution reaction . It can also convert to the oxide when heated (calcined) .Physical And Chemical Properties Analysis
Nickel(II) oxalate hydrate is a green crystalline powder . It is highly insoluble in water and converts to the oxide when heated (calcined) . The carbon–carbon bond in its structure is the weakest one .Scientific Research Applications
Electrochemical Applications
Nickel(II) oxalate hydrate can be used in the development of non-precious electrocatalysts for improved alkaline oxygen evolution reactions . The nickel-cobalt oxalate (Ni2.5Co5C2O4) developed using this compound was found to have superior performance compared to other catalysts like NiC2O4, CoC2O4, NiCo2O4, and RuO2 .
Energy Storage
Nickel(II) oxalate hydrate can be used in the synthesis of NiS2 nanocubes, which have been shown to be effective as electrodes for high-performance supercapacitors . The NiS2 nanocubes demonstrated excellent electrochemical performance with high specific capacitance, low resistance, and excellent cycling stability .
Organic Synthesis
Nickel(II) oxalate hydrate is an important raw material and intermediate used in organic synthesis . It can be used in the synthesis of various organic compounds.
Pharmaceuticals
This compound is also used in the pharmaceutical industry . It can be used in the synthesis of various pharmaceutical compounds.
Agrochemicals
Nickel(II) oxalate hydrate is used in the agrochemical industry . It can be used in the synthesis of various agrochemicals.
Dyestuffs
This compound is used in the dyestuff industry . It can be used in the synthesis of various dyes.
Mechanism of Action
Target of Action
Nickel(II) oxalate hydrate primarily targets the oxygen evolution reaction (OER) in an alkaline medium . The compound acts as an efficient non-precious electrocatalyst, contributing to the development of next-generation electrocatalysts .
Mode of Action
Nickel(II) oxalate hydrate interacts with its targets through a proton decoupled electron transfer (PDET) mechanism . This interaction leads to changes in the overpotential required for the OER, with the compound exhibiting a lower charge transfer resistance and impressive stability performance .
Biochemical Pathways
The compound affects the alkaline oxygen evolution reaction (OER) pathway . Its action results in an improved electrochemical activity, attributed to double layer capacitance (Cdl), which highlights the superiority of Nickel(II) oxalate hydrate over other compounds like NiCo2O4 for the alkaline OER .
Result of Action
The action of Nickel(II) oxalate hydrate leads to the formation of a flake-like porous nanostructure, indicating a distinct transformation in morphology during the alkaline OER process . XPS analysis demonstrates complete oxidation of Ni2+ and Co2+ centres into Ni3+ and Co3+, respectively, under high oxidation potential, thereby indicating active site formation throughout the microstructural network .
Action Environment
The action, efficacy, and stability of Nickel(II) oxalate hydrate are influenced by environmental factors such as the pH of the medium and the reaction temperature . For instance, the compound exhibits 87% faradaic efficiency in an alkaline medium (pH 14) . The synthetic parameters for the preparation of the compound, such as the metal precursor (Ni/Co) ratio, oxalic acid amount, reaction temperature, and time, are optimized to enhance its performance .
Safety and Hazards
Future Directions
properties
IUPAC Name |
nickel(2+);oxalate;hydrate | |
---|---|---|
Source | PubChem | |
URL | https://pubchem.ncbi.nlm.nih.gov | |
Description | Data deposited in or computed by PubChem | |
InChI |
InChI=1S/C2H2O4.Ni.H2O/c3-1(4)2(5)6;;/h(H,3,4)(H,5,6);;1H2/q;+2;/p-2 | |
Source | PubChem | |
URL | https://pubchem.ncbi.nlm.nih.gov | |
Description | Data deposited in or computed by PubChem | |
InChI Key |
DCEDPLWSSQQNGQ-UHFFFAOYSA-L | |
Source | PubChem | |
URL | https://pubchem.ncbi.nlm.nih.gov | |
Description | Data deposited in or computed by PubChem | |
Canonical SMILES |
C(=O)(C(=O)[O-])[O-].O.[Ni+2] | |
Source | PubChem | |
URL | https://pubchem.ncbi.nlm.nih.gov | |
Description | Data deposited in or computed by PubChem | |
Molecular Formula |
C2H2NiO5 | |
Source | PubChem | |
URL | https://pubchem.ncbi.nlm.nih.gov | |
Description | Data deposited in or computed by PubChem | |
DSSTOX Substance ID |
DTXSID30583775 | |
Record name | Nickel(2+) ethanedioate--water (1/1/1) | |
Source | EPA DSSTox | |
URL | https://comptox.epa.gov/dashboard/DTXSID30583775 | |
Description | DSSTox provides a high quality public chemistry resource for supporting improved predictive toxicology. | |
Molecular Weight |
164.73 g/mol | |
Source | PubChem | |
URL | https://pubchem.ncbi.nlm.nih.gov | |
Description | Data deposited in or computed by PubChem | |
Product Name |
Nickel(II) oxalate hydrate | |
CAS RN |
126956-48-7 | |
Record name | Nickel(2+) ethanedioate--water (1/1/1) | |
Source | EPA DSSTox | |
URL | https://comptox.epa.gov/dashboard/DTXSID30583775 | |
Description | DSSTox provides a high quality public chemistry resource for supporting improved predictive toxicology. | |
Retrosynthesis Analysis
AI-Powered Synthesis Planning: Our tool employs the Template_relevance Pistachio, Template_relevance Bkms_metabolic, Template_relevance Pistachio_ringbreaker, Template_relevance Reaxys, Template_relevance Reaxys_biocatalysis model, leveraging a vast database of chemical reactions to predict feasible synthetic routes.
One-Step Synthesis Focus: Specifically designed for one-step synthesis, it provides concise and direct routes for your target compounds, streamlining the synthesis process.
Accurate Predictions: Utilizing the extensive PISTACHIO, BKMS_METABOLIC, PISTACHIO_RINGBREAKER, REAXYS, REAXYS_BIOCATALYSIS database, our tool offers high-accuracy predictions, reflecting the latest in chemical research and data.
Strategy Settings
Precursor scoring | Relevance Heuristic |
---|---|
Min. plausibility | 0.01 |
Model | Template_relevance |
Template Set | Pistachio/Bkms_metabolic/Pistachio_ringbreaker/Reaxys/Reaxys_biocatalysis |
Top-N result to add to graph | 6 |
Feasible Synthetic Routes
Disclaimer and Information on In-Vitro Research Products
Please be aware that all articles and product information presented on BenchChem are intended solely for informational purposes. The products available for purchase on BenchChem are specifically designed for in-vitro studies, which are conducted outside of living organisms. In-vitro studies, derived from the Latin term "in glass," involve experiments performed in controlled laboratory settings using cells or tissues. It is important to note that these products are not categorized as medicines or drugs, and they have not received approval from the FDA for the prevention, treatment, or cure of any medical condition, ailment, or disease. We must emphasize that any form of bodily introduction of these products into humans or animals is strictly prohibited by law. It is essential to adhere to these guidelines to ensure compliance with legal and ethical standards in research and experimentation.