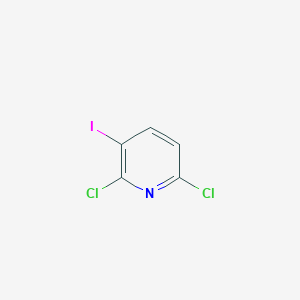
2,6-Dichloro-3-iodopyridine
Overview
Description
2,6-Dichloro-3-iodopyridine is an organic compound with the molecular formula C5H2Cl2IN It is a derivative of pyridine, characterized by the presence of two chlorine atoms and one iodine atom attached to the pyridine ring
Preparation Methods
Synthetic Routes and Reaction Conditions: The synthesis of 2,6-Dichloro-3-iodopyridine typically involves halogenation reactions. One common method includes the reaction of 2,6-dichloropyridine with iodine in the presence of a suitable catalyst. The reaction is usually carried out in an organic solvent such as tetrahydrofuran or dimethylformamide, under controlled temperature conditions ranging from room temperature to 50°C .
Industrial Production Methods: Industrial production of this compound follows similar synthetic routes but on a larger scale. The process involves the use of high-purity reagents and optimized reaction conditions to ensure maximum yield and purity. The product is then purified through crystallization or distillation techniques.
Chemical Reactions Analysis
Types of Reactions: 2,6-Dichloro-3-iodopyridine undergoes various types of chemical reactions, including:
Substitution Reactions: The iodine atom can be replaced by other nucleophiles in substitution reactions.
Oxidation and Reduction Reactions: The compound can participate in redox reactions, altering its oxidation state.
Coupling Reactions: It can be used in cross-coupling reactions such as Suzuki and Stille couplings, where it forms carbon-carbon bonds with other organic molecules.
Common Reagents and Conditions:
Substitution Reactions: Common reagents include potassium fluoride and copper(I) iodide.
Coupling Reactions: Palladium catalysts are often used in Suzuki and Stille couplings.
Major Products: The major products formed from these reactions depend on the specific reagents and conditions used. For example, in a Suzuki coupling reaction, the product would be a biaryl compound.
Scientific Research Applications
2,6-Dichloro-3-iodopyridine has a wide range of applications in scientific research:
Chemistry: It is used as a building block in the synthesis of more complex organic molecules.
Biology: The compound is used in the development of bioactive molecules and pharmaceuticals.
Medicine: It serves as an intermediate in the synthesis of drugs and therapeutic agents.
Industry: It is used in the production of agrochemicals and other industrial chemicals.
Mechanism of Action
The mechanism of action of 2,6-Dichloro-3-iodopyridine involves its ability to participate in various chemical reactions due to the presence of reactive halogen atoms. These atoms can undergo substitution, coupling, and other reactions, making the compound a versatile intermediate in organic synthesis. The molecular targets and pathways involved depend on the specific application and the nature of the reaction .
Comparison with Similar Compounds
- 2,6-Dichloro-4-iodopyridine
- 2,6-Dichloro-3-methoxypyridine
- 2,6-Dichloro-N,N-dimethylpyridin-4-amine
Comparison: 2,6-Dichloro-3-iodopyridine is unique due to the specific positioning of the chlorine and iodine atoms on the pyridine ring. This unique arrangement imparts distinct reactivity and properties compared to its analogs. For instance, the presence of iodine at the 3-position makes it particularly suitable for coupling reactions, whereas other derivatives might be more reactive in different types of chemical transformations .
Properties
IUPAC Name |
2,6-dichloro-3-iodopyridine | |
---|---|---|
Source | PubChem | |
URL | https://pubchem.ncbi.nlm.nih.gov | |
Description | Data deposited in or computed by PubChem | |
InChI |
InChI=1S/C5H2Cl2IN/c6-4-2-1-3(8)5(7)9-4/h1-2H | |
Source | PubChem | |
URL | https://pubchem.ncbi.nlm.nih.gov | |
Description | Data deposited in or computed by PubChem | |
InChI Key |
DPCQIHCGMIPSQV-UHFFFAOYSA-N | |
Source | PubChem | |
URL | https://pubchem.ncbi.nlm.nih.gov | |
Description | Data deposited in or computed by PubChem | |
Canonical SMILES |
C1=CC(=NC(=C1I)Cl)Cl | |
Source | PubChem | |
URL | https://pubchem.ncbi.nlm.nih.gov | |
Description | Data deposited in or computed by PubChem | |
Molecular Formula |
C5H2Cl2IN | |
Source | PubChem | |
URL | https://pubchem.ncbi.nlm.nih.gov | |
Description | Data deposited in or computed by PubChem | |
DSSTOX Substance ID |
DTXSID10371076 | |
Record name | 2,6-Dichloro-3-iodopyridine | |
Source | EPA DSSTox | |
URL | https://comptox.epa.gov/dashboard/DTXSID10371076 | |
Description | DSSTox provides a high quality public chemistry resource for supporting improved predictive toxicology. | |
Molecular Weight |
273.88 g/mol | |
Source | PubChem | |
URL | https://pubchem.ncbi.nlm.nih.gov | |
Description | Data deposited in or computed by PubChem | |
CAS No. |
148493-37-2 | |
Record name | 2,6-Dichloro-3-iodopyridine | |
Source | EPA DSSTox | |
URL | https://comptox.epa.gov/dashboard/DTXSID10371076 | |
Description | DSSTox provides a high quality public chemistry resource for supporting improved predictive toxicology. | |
Record name | 2,6-Dichloro-3-iodopyridine | |
Source | European Chemicals Agency (ECHA) | |
URL | https://echa.europa.eu/information-on-chemicals | |
Description | The European Chemicals Agency (ECHA) is an agency of the European Union which is the driving force among regulatory authorities in implementing the EU's groundbreaking chemicals legislation for the benefit of human health and the environment as well as for innovation and competitiveness. | |
Explanation | Use of the information, documents and data from the ECHA website is subject to the terms and conditions of this Legal Notice, and subject to other binding limitations provided for under applicable law, the information, documents and data made available on the ECHA website may be reproduced, distributed and/or used, totally or in part, for non-commercial purposes provided that ECHA is acknowledged as the source: "Source: European Chemicals Agency, http://echa.europa.eu/". Such acknowledgement must be included in each copy of the material. ECHA permits and encourages organisations and individuals to create links to the ECHA website under the following cumulative conditions: Links can only be made to webpages that provide a link to the Legal Notice page. | |
Retrosynthesis Analysis
AI-Powered Synthesis Planning: Our tool employs the Template_relevance Pistachio, Template_relevance Bkms_metabolic, Template_relevance Pistachio_ringbreaker, Template_relevance Reaxys, Template_relevance Reaxys_biocatalysis model, leveraging a vast database of chemical reactions to predict feasible synthetic routes.
One-Step Synthesis Focus: Specifically designed for one-step synthesis, it provides concise and direct routes for your target compounds, streamlining the synthesis process.
Accurate Predictions: Utilizing the extensive PISTACHIO, BKMS_METABOLIC, PISTACHIO_RINGBREAKER, REAXYS, REAXYS_BIOCATALYSIS database, our tool offers high-accuracy predictions, reflecting the latest in chemical research and data.
Strategy Settings
Precursor scoring | Relevance Heuristic |
---|---|
Min. plausibility | 0.01 |
Model | Template_relevance |
Template Set | Pistachio/Bkms_metabolic/Pistachio_ringbreaker/Reaxys/Reaxys_biocatalysis |
Top-N result to add to graph | 6 |
Feasible Synthetic Routes
Disclaimer and Information on In-Vitro Research Products
Please be aware that all articles and product information presented on BenchChem are intended solely for informational purposes. The products available for purchase on BenchChem are specifically designed for in-vitro studies, which are conducted outside of living organisms. In-vitro studies, derived from the Latin term "in glass," involve experiments performed in controlled laboratory settings using cells or tissues. It is important to note that these products are not categorized as medicines or drugs, and they have not received approval from the FDA for the prevention, treatment, or cure of any medical condition, ailment, or disease. We must emphasize that any form of bodily introduction of these products into humans or animals is strictly prohibited by law. It is essential to adhere to these guidelines to ensure compliance with legal and ethical standards in research and experimentation.