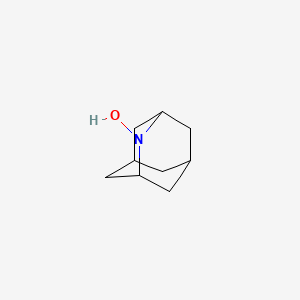
2-Hydroxy-2-azaadamantane
Overview
Description
2-Hydroxy-2-azaadamantane is a nitrogen-containing analog of adamantane, characterized by the presence of a hydroxyl group and a nitrogen atom within its adamantane framework
Mechanism of Action
Target of Action
The primary target of 2-Hydroxy-2-azaadamantane is the C (sp3)-H bond in organic compounds . This compound acts as a catalyst for the efficient functionalization of this bond .
Mode of Action
This compound interacts with its target by catalyzing the oxidation of alcohols . It has been reported that this compound, also known as AZADOL®, exhibits excellent catalytic activity, enabling the oxidation of a variety of alcohols . This catalytic reaction undergoes the mechanism of 2-azadamantane-N-oxygen (AZADO), so that this compound presents the same properties as AZADO .
Biochemical Pathways
The primary biochemical pathway affected by this compound is the oxidation of alcohols to their corresponding aldehydes or ketones . This transformation is fundamental in organic synthesis . The highly active nature of this compound has spurred further use in alcohol oxidations .
Pharmacokinetics
It is known that this compound has better storage stability than azado . More research is needed to fully understand the ADME properties of this compound and their impact on bioavailability.
Result of Action
The result of the action of this compound is the efficient oxidation of alcohols to their corresponding aldehydes or ketones . This compound has been found to exhibit excellent catalytic activity, enabling the oxidation of a variety of alcohols .
Action Environment
The action of this compound is influenced by the presence of an oxidizing agent . In the presence of such an agent, the catalytic reaction undergoes the mechanism of AZADO . The storage conditions can also influence the stability of this compound . It is recommended to store this compound at room temperature, preferably in a cool and dark place .
Biochemical Analysis
Biochemical Properties
2-Hydroxy-2-azaadamantane is a catalyst for the efficient C (sp3)-H bond functionalization of Isochroman . It has been shown that this compound can be synthesized from primary alcohols, aldehydes, and celluloses .
Cellular Effects
It is known that it has a potent antitumor activity .
Molecular Mechanism
The molecular mechanism of this compound involves the oxidation of α-hydroxy acids to α-keto acids . This process is catalyzed by 2-azaadamantane N-oxyl (AZADO), a nitroxyl radical catalyst . The use of molecular oxygen as a cooxidant enables the desired chemoselective oxidation to α-keto acids .
Temporal Effects in Laboratory Settings
It is known that the reaction time of this compound is fast and the reaction products are stable .
Preparation Methods
Synthetic Routes and Reaction Conditions: The synthesis of 2-Hydroxy-2-azaadamantane typically involves the introduction of a nitrogen atom into the adamantane structure followed by the addition of a hydroxyl group. One common method includes the reaction of 2-azaadamantane with an oxidizing agent to introduce the hydroxyl group. The reaction conditions often involve the use of mild oxidizing agents to ensure selective hydroxylation without over-oxidation.
Industrial Production Methods: Industrial production of this compound may involve large-scale synthesis using optimized reaction conditions to maximize yield and purity. The process typically includes steps such as purification and crystallization to obtain the compound in its desired form.
Types of Reactions:
Oxidation: this compound can undergo oxidation reactions, often catalyzed by nitroxyl radicals, to form oxoammonium salts.
Reduction: The compound can be reduced under specific conditions to yield various reduced forms.
Substitution: It can participate in substitution reactions where the hydroxyl group is replaced by other functional groups.
Common Reagents and Conditions:
Oxidation: Common oxidizing agents include sodium hypochlorite and iodobenzene diacetate.
Reduction: Reducing agents such as sodium borohydride can be used.
Substitution: Conditions vary depending on the substituent being introduced, but often involve the use of catalysts and specific solvents.
Major Products:
Oxidation: Formation of oxoammonium salts.
Reduction: Various reduced derivatives of this compound.
Substitution: Substituted azaadamantane derivatives with different functional groups.
Scientific Research Applications
2-Hydroxy-2-azaadamantane has found applications in several scientific fields:
Chemistry: Used as a catalyst in oxidation reactions, particularly for the oxidation of alcohols to aldehydes or ketones.
Biology: Investigated for its potential biological activity, including antiviral and antitumor properties.
Medicine: Explored for its potential use in drug development due to its unique structural properties.
Industry: Utilized in the synthesis of various organic compounds and as a building block for more complex molecules.
Comparison with Similar Compounds
2-Azaadamantane: Lacks the hydroxyl group but shares the nitrogen-containing adamantane structure.
1,3-Dimethyl-2-azaadamantane: Contains additional methyl groups, altering its reactivity and properties.
9-Azanoradamantane: Another nitrogen-containing analog with a different structural arrangement.
Uniqueness: 2-Hydroxy-2-azaadamantane is unique due to the presence of both a nitrogen atom and a hydroxyl group within the adamantane framework. This combination imparts distinct chemical reactivity and potential for diverse applications, setting it apart from other similar compounds.
Properties
IUPAC Name |
2-hydroxy-2-azatricyclo[3.3.1.13,7]decane | |
---|---|---|
Source | PubChem | |
URL | https://pubchem.ncbi.nlm.nih.gov | |
Description | Data deposited in or computed by PubChem | |
InChI |
InChI=1S/C9H15NO/c11-10-8-2-6-1-7(4-8)5-9(10)3-6/h6-9,11H,1-5H2 | |
Source | PubChem | |
URL | https://pubchem.ncbi.nlm.nih.gov | |
Description | Data deposited in or computed by PubChem | |
InChI Key |
SFXINVPZIZMFHM-UHFFFAOYSA-N | |
Source | PubChem | |
URL | https://pubchem.ncbi.nlm.nih.gov | |
Description | Data deposited in or computed by PubChem | |
Canonical SMILES |
C1C2CC3CC1CC(C2)N3O | |
Source | PubChem | |
URL | https://pubchem.ncbi.nlm.nih.gov | |
Description | Data deposited in or computed by PubChem | |
Molecular Formula |
C9H15NO | |
Source | PubChem | |
URL | https://pubchem.ncbi.nlm.nih.gov | |
Description | Data deposited in or computed by PubChem | |
Molecular Weight |
153.22 g/mol | |
Source | PubChem | |
URL | https://pubchem.ncbi.nlm.nih.gov | |
Description | Data deposited in or computed by PubChem | |
Retrosynthesis Analysis
AI-Powered Synthesis Planning: Our tool employs the Template_relevance Pistachio, Template_relevance Bkms_metabolic, Template_relevance Pistachio_ringbreaker, Template_relevance Reaxys, Template_relevance Reaxys_biocatalysis model, leveraging a vast database of chemical reactions to predict feasible synthetic routes.
One-Step Synthesis Focus: Specifically designed for one-step synthesis, it provides concise and direct routes for your target compounds, streamlining the synthesis process.
Accurate Predictions: Utilizing the extensive PISTACHIO, BKMS_METABOLIC, PISTACHIO_RINGBREAKER, REAXYS, REAXYS_BIOCATALYSIS database, our tool offers high-accuracy predictions, reflecting the latest in chemical research and data.
Strategy Settings
Precursor scoring | Relevance Heuristic |
---|---|
Min. plausibility | 0.01 |
Model | Template_relevance |
Template Set | Pistachio/Bkms_metabolic/Pistachio_ringbreaker/Reaxys/Reaxys_biocatalysis |
Top-N result to add to graph | 6 |
Feasible Synthetic Routes
Q1: How does 2-hydroxy-2-azaadamantane contribute to understanding the reactivity of cobalt(III)-superoxido complexes?
A: this compound (AZADOL) serves as a hydrogen atom donor in this study []. Researchers used AZADOL to investigate how effectively cobalt(III)-superoxido complexes, stabilized by different ligands, could abstract a hydrogen atom (H-atom). This process mimics a crucial step in the conversion of superoxide (O2−) to hydroperoxide (OOH−) by certain enzymes. By analyzing the rate of reaction between the cobalt(III)-superoxido complexes and AZADOL, researchers gained insights into how the electronic and structural properties of the supporting ligands influence the reactivity of the superoxide species bound to the cobalt(III) center [].
Disclaimer and Information on In-Vitro Research Products
Please be aware that all articles and product information presented on BenchChem are intended solely for informational purposes. The products available for purchase on BenchChem are specifically designed for in-vitro studies, which are conducted outside of living organisms. In-vitro studies, derived from the Latin term "in glass," involve experiments performed in controlled laboratory settings using cells or tissues. It is important to note that these products are not categorized as medicines or drugs, and they have not received approval from the FDA for the prevention, treatment, or cure of any medical condition, ailment, or disease. We must emphasize that any form of bodily introduction of these products into humans or animals is strictly prohibited by law. It is essential to adhere to these guidelines to ensure compliance with legal and ethical standards in research and experimentation.