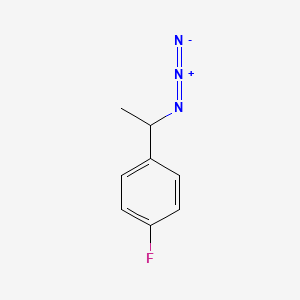
1-(1-Azidoethyl)-4-fluorobenzene
- Click on QUICK INQUIRY to receive a quote from our team of experts.
- With the quality product at a COMPETITIVE price, you can focus more on your research.
Overview
Description
1-(1-Azidoethyl)-4-fluorobenzene is an organic compound characterized by the presence of an azido group (-N₃) attached to an ethyl group, which is further connected to a benzene ring substituted with a fluorine atom
Preparation Methods
The synthesis of 1-(1-Azidoethyl)-4-fluorobenzene typically involves the azidation of an appropriate precursor. One common method is the nucleophilic substitution reaction where a halogenated precursor, such as 1-(1-bromoethyl)-4-fluorobenzene, reacts with sodium azide (NaN₃) in a polar aprotic solvent like dimethyl sulfoxide (DMSO). The reaction conditions often include moderate temperatures and stirring to ensure complete conversion .
Industrial production methods may involve similar synthetic routes but on a larger scale, with optimizations for yield and purity. The use of continuous flow reactors and automated systems can enhance the efficiency and scalability of the process .
Chemical Reactions Analysis
1-(1-Azidoethyl)-4-fluorobenzene undergoes various chemical reactions, including:
Oxidation: The azido group can be oxidized to form nitro compounds under specific conditions.
Reduction: Reduction of the azido group can yield amines, which are valuable intermediates in organic synthesis.
Substitution: The azido group can participate in substitution reactions, such as the Staudinger reaction, to form phosphorimidates.
Common reagents used in these reactions include reducing agents like lithium aluminum hydride (LiAlH₄) for reductions, and copper catalysts for cycloaddition reactions. The major products formed depend on the specific reaction conditions and reagents used.
Scientific Research Applications
1-(1-Azidoethyl)-4-fluorobenzene has diverse applications in scientific research:
Chemistry: It serves as a precursor for the synthesis of various nitrogen-containing heterocycles and other complex organic molecules.
Biology: The compound can be used in bioorthogonal chemistry for labeling and tracking biomolecules in living systems.
Industry: It is used in the synthesis of advanced materials, including polymers and explosives
Mechanism of Action
The mechanism by which 1-(1-Azidoethyl)-4-fluorobenzene exerts its effects is primarily through the reactivity of the azido group. The azido group can undergo various transformations, such as reduction to amines or cycloaddition to form triazoles. These reactions often involve the formation of reactive intermediates, such as nitrenes, which can further react with other molecules .
Comparison with Similar Compounds
1-(1-Azidoethyl)-4-fluorobenzene can be compared with other azido compounds, such as:
1-(Azidomethyl)-5H-tetrazole: Known for its high energy content and use in energetic materials.
1-Azidoethyl-5H-tetrazole: Similar in structure but with different reactivity and applications.
1-Azidoethyl-choline: Used in the synthesis of choline-containing phospholipids.
The uniqueness of this compound lies in its specific substitution pattern, which imparts distinct chemical properties and reactivity compared to other azido compounds.
Properties
CAS No. |
823189-13-5 |
---|---|
Molecular Formula |
C8H8FN3 |
Molecular Weight |
165.17 g/mol |
IUPAC Name |
1-(1-azidoethyl)-4-fluorobenzene |
InChI |
InChI=1S/C8H8FN3/c1-6(11-12-10)7-2-4-8(9)5-3-7/h2-6H,1H3 |
InChI Key |
CDHHTJIEVYLEFO-UHFFFAOYSA-N |
Canonical SMILES |
CC(C1=CC=C(C=C1)F)N=[N+]=[N-] |
Origin of Product |
United States |
Disclaimer and Information on In-Vitro Research Products
Please be aware that all articles and product information presented on BenchChem are intended solely for informational purposes. The products available for purchase on BenchChem are specifically designed for in-vitro studies, which are conducted outside of living organisms. In-vitro studies, derived from the Latin term "in glass," involve experiments performed in controlled laboratory settings using cells or tissues. It is important to note that these products are not categorized as medicines or drugs, and they have not received approval from the FDA for the prevention, treatment, or cure of any medical condition, ailment, or disease. We must emphasize that any form of bodily introduction of these products into humans or animals is strictly prohibited by law. It is essential to adhere to these guidelines to ensure compliance with legal and ethical standards in research and experimentation.