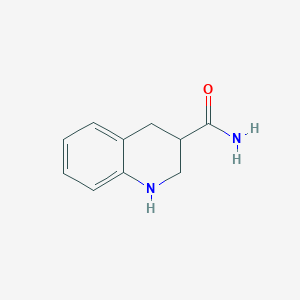
1,2,3,4-Tetrahydroquinoline-3-carboxamide
Overview
Description
1,2,3,4-Tetrahydroquinoline-3-carboxamide is a heterocyclic organic compound that belongs to the class of tetrahydroquinolines. These compounds are known for their diverse biological activities and have garnered significant interest in medicinal chemistry. The structure of this compound consists of a quinoline ring system that is partially saturated, making it a versatile scaffold for various chemical modifications and applications .
Mechanism of Action
Target of Action
The primary target of 1,2,3,4-Tetrahydroquinoline-3-carboxamide is the retinoic acid receptor-related orphan receptor γ (RORγ) . RORγ is a nuclear receptor that plays a crucial role in energy homeostasis and immuno-oncology . It is regarded as an attractive therapeutic target for the treatment of various cancers, including prostate cancer .
Mode of Action
This compound acts as an inverse agonist of RORγ . It effectively inhibits the RORγ transcriptional activity and exhibits excellent selectivity against other nuclear receptor subtypes . The compound induces allosteric modulation, causing a conformational change in the enzyme that prevents AMP from binding .
Biochemical Pathways
The compound’s action on RORγ affects the differentiation of CD4+T cells into Th17 cells and the production of pro-inflammatory cytokines, including IL-17 (interleukin 17) and IL-22 . This direct impact on the Th17/IL-17 pathway makes RORγ an attractive drug target for the treatment of autoimmune diseases, particularly psoriasis .
Pharmacokinetics
These compounds also effectively migrate through biological membranes by means of passive diffusion .
Result of Action
The action of this compound results in potent inhibition of colony formation and the expression of AR, AR regulated genes, and other oncogenes in AR positive prostate cancer cell lines . Moreover, it effectively suppresses tumor growth in a 22Rv1 xenograft tumor model in mice .
Action Environment
Environmental factors can influence the action, efficacy, and stability of this compound. For instance, the compound is thermally stable above 200 °C , suggesting that it can maintain its efficacy under a range of temperatures.
Biochemical Analysis
Biochemical Properties
Tetrahydroquinoline analogs are known to exert diverse biological activities against various infective pathogens and neurodegenerative disorders
Molecular Mechanism
It is likely that the compound exerts its effects at the molecular level through binding interactions with biomolecules, enzyme inhibition or activation, and changes in gene expression
Preparation Methods
Synthetic Routes and Reaction Conditions: 1,2,3,4-Tetrahydroquinoline-3-carboxamide can be synthesized through several methods. One common approach involves the reduction of quinoline derivatives. For instance, the
Properties
IUPAC Name |
1,2,3,4-tetrahydroquinoline-3-carboxamide | |
---|---|---|
Source | PubChem | |
URL | https://pubchem.ncbi.nlm.nih.gov | |
Description | Data deposited in or computed by PubChem | |
InChI |
InChI=1S/C10H12N2O/c11-10(13)8-5-7-3-1-2-4-9(7)12-6-8/h1-4,8,12H,5-6H2,(H2,11,13) | |
Source | PubChem | |
URL | https://pubchem.ncbi.nlm.nih.gov | |
Description | Data deposited in or computed by PubChem | |
InChI Key |
NYENCZIONJUOSQ-UHFFFAOYSA-N | |
Source | PubChem | |
URL | https://pubchem.ncbi.nlm.nih.gov | |
Description | Data deposited in or computed by PubChem | |
Canonical SMILES |
C1C(CNC2=CC=CC=C21)C(=O)N | |
Source | PubChem | |
URL | https://pubchem.ncbi.nlm.nih.gov | |
Description | Data deposited in or computed by PubChem | |
Molecular Formula |
C10H12N2O | |
Source | PubChem | |
URL | https://pubchem.ncbi.nlm.nih.gov | |
Description | Data deposited in or computed by PubChem | |
DSSTOX Substance ID |
DTXSID701261462 | |
Record name | 3-Quinolinecarboxamide, 1,2,3,4-tetrahydro- | |
Source | EPA DSSTox | |
URL | https://comptox.epa.gov/dashboard/DTXSID701261462 | |
Description | DSSTox provides a high quality public chemistry resource for supporting improved predictive toxicology. | |
Molecular Weight |
176.21 g/mol | |
Source | PubChem | |
URL | https://pubchem.ncbi.nlm.nih.gov | |
Description | Data deposited in or computed by PubChem | |
CAS No. |
4138-23-2 | |
Record name | 3-Quinolinecarboxamide, 1,2,3,4-tetrahydro- | |
Source | CAS Common Chemistry | |
URL | https://commonchemistry.cas.org/detail?cas_rn=4138-23-2 | |
Description | CAS Common Chemistry is an open community resource for accessing chemical information. Nearly 500,000 chemical substances from CAS REGISTRY cover areas of community interest, including common and frequently regulated chemicals, and those relevant to high school and undergraduate chemistry classes. This chemical information, curated by our expert scientists, is provided in alignment with our mission as a division of the American Chemical Society. | |
Explanation | The data from CAS Common Chemistry is provided under a CC-BY-NC 4.0 license, unless otherwise stated. | |
Record name | 3-Quinolinecarboxamide, 1,2,3,4-tetrahydro- | |
Source | EPA DSSTox | |
URL | https://comptox.epa.gov/dashboard/DTXSID701261462 | |
Description | DSSTox provides a high quality public chemistry resource for supporting improved predictive toxicology. | |
Retrosynthesis Analysis
AI-Powered Synthesis Planning: Our tool employs the Template_relevance Pistachio, Template_relevance Bkms_metabolic, Template_relevance Pistachio_ringbreaker, Template_relevance Reaxys, Template_relevance Reaxys_biocatalysis model, leveraging a vast database of chemical reactions to predict feasible synthetic routes.
One-Step Synthesis Focus: Specifically designed for one-step synthesis, it provides concise and direct routes for your target compounds, streamlining the synthesis process.
Accurate Predictions: Utilizing the extensive PISTACHIO, BKMS_METABOLIC, PISTACHIO_RINGBREAKER, REAXYS, REAXYS_BIOCATALYSIS database, our tool offers high-accuracy predictions, reflecting the latest in chemical research and data.
Strategy Settings
Precursor scoring | Relevance Heuristic |
---|---|
Min. plausibility | 0.01 |
Model | Template_relevance |
Template Set | Pistachio/Bkms_metabolic/Pistachio_ringbreaker/Reaxys/Reaxys_biocatalysis |
Top-N result to add to graph | 6 |
Feasible Synthetic Routes
Q1: What are the key structural features of 1,2,3,4-Tetrahydroquinoline-3-carboxamides and how are they synthesized?
A1: 1,2,3,4-Tetrahydroquinoline-3-carboxamides are characterized by a tetrahydroquinoline ring system with a carboxamide substituent at the 3-position. Recent research focuses on their enantioselective synthesis through copper-catalyzed intramolecular aryl C-N coupling reactions. [, ] This method utilizes readily available malonamide derivatives as starting materials and employs a desymmetrization strategy to differentiate the two amide groups. [, ] Researchers have explored various chiral ligands and reaction conditions to optimize yield and enantioselectivity. [, ]
Q2: How does the structure of the malonamide starting material influence the stereochemical outcome of the reaction?
A2: Studies have shown that the substituents on the malonamide nitrogen atoms play a crucial role in controlling the enantioselectivity of the copper-catalyzed cyclization. [] For instance, bulky groups on the nitrogen, such as tert-butylphenyl, can restrict rotation and lead to higher diastereoselectivity during the formation of the chiral axis in the product. [] Additionally, the chirality of the α-carbon in the malonamide substrate can influence the stereochemical outcome of the reaction, suggesting a degree of substrate control over the formation of the chiral axis in the product. []
Q3: Beyond copper catalysis, what other synthetic approaches have been investigated for the synthesis of these compounds?
A3: While copper-catalyzed C-N coupling has been a focus, researchers have also investigated alternative methods for constructing the tetrahydroquinoline core. One approach utilizes a palladium-catalyzed Buchwald-Hartwig reaction for intramolecular cyclization. [, ] This method has shown promise in achieving enantioselectivity with the appropriate chiral ligands. [, ] Furthermore, Goldberg reactions have been employed to generate 5-membered rings from malonamide derivatives bearing a pendant bromovinyl substituent. []
Q4: What are the potential applications of enantioenriched 1,2,3,4-Tetrahydroquinoline-3-carboxamides?
A4: While the explored research primarily focuses on synthetic methodology, the enantioselective synthesis of these compounds holds promise for various applications. The tetrahydroquinoline scaffold is a prevalent structural motif in numerous biologically active natural products and pharmaceuticals. [] Therefore, access to enantioenriched 1,2,3,4-Tetrahydroquinoline-3-carboxamides could facilitate the development of new pharmaceuticals with improved pharmacological properties and potentially reduced side effects.
Disclaimer and Information on In-Vitro Research Products
Please be aware that all articles and product information presented on BenchChem are intended solely for informational purposes. The products available for purchase on BenchChem are specifically designed for in-vitro studies, which are conducted outside of living organisms. In-vitro studies, derived from the Latin term "in glass," involve experiments performed in controlled laboratory settings using cells or tissues. It is important to note that these products are not categorized as medicines or drugs, and they have not received approval from the FDA for the prevention, treatment, or cure of any medical condition, ailment, or disease. We must emphasize that any form of bodily introduction of these products into humans or animals is strictly prohibited by law. It is essential to adhere to these guidelines to ensure compliance with legal and ethical standards in research and experimentation.