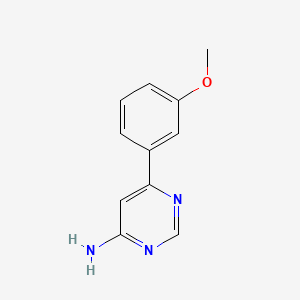
6-(3-Methoxyphenyl)pyrimidin-4-amine
Overview
Description
“6-(3-Methoxyphenyl)pyrimidin-4-amine” is a pyrimidine derivative . Pyrimidines are aromatic heterocyclic compounds that contain two nitrogen atoms at positions 1 and 3 of the six-membered ring . They display a range of pharmacological effects including antioxidants, antibacterial, antiviral, antifungal, antituberculosis, and anti-inflammatory .
Scientific Research Applications
Anticancer Properties
Pyrimidine derivatives, including those similar to 6-(3-Methoxyphenyl)pyrimidin-4-amine, have been studied for their potential anticancer properties. Some compounds in this class have shown inhibitory effects on cell proliferation, which is a key factor in cancer treatment strategies .
Anti-inflammatory Activities
The anti-inflammatory effects of pyrimidines are attributed to their ability to inhibit the expression and activities of vital inflammatory mediators such as prostaglandin E2, inducible nitric oxide synthase, tumor necrosis factor-α, nuclear factor κB, leukotrienes, and some interleukins .
Corrosion Inhibition
Nitrogen-based heterocyclic compounds like pyrimidines are extensively used as corrosion inhibitors due to their strong coordination bonding capabilities with metallic atoms. This application is crucial in protecting materials and infrastructure from degradation.
Neuroprotective Effects
Pyrimidine pharmacophores have shown a broad spectrum of biological activities, including potential use against neurodegenerative diseases such as Parkinson’s disease. This suggests that derivatives like 6-(3-Methoxyphenyl)pyrimidin-4-amine could have applications in neuroprotection .
Microwave-Assisted Synthesis
The compound has been utilized in microwave-assisted synthetic processes, which are known for their efficiency and rapid reaction times. This application is significant in the field of pharmaceuticals where time and resource efficiency are critical .
Mechanism of Action
Target of Action
Similar compounds such as 2-aminopyrimidine derivatives have shown activity against organisms causing diseases like sleeping sickness and malaria .
Mode of Action
It’s worth noting that similar compounds, such as 2-aminopyrimidine derivatives, have shown to exhibit antitrypanosomal and antiplasmodial activities . These compounds interact with their targets, leading to changes that inhibit the growth and proliferation of the disease-causing organisms .
Biochemical Pathways
Related compounds have been shown to influence pathways related to inflammation and cellular signaling .
Result of Action
Similar compounds have shown to exhibit antitrypanosomal and antiplasmodial activities, indicating their potential to inhibit the growth and proliferation of disease-causing organisms .
properties
IUPAC Name |
6-(3-methoxyphenyl)pyrimidin-4-amine | |
---|---|---|
Source | PubChem | |
URL | https://pubchem.ncbi.nlm.nih.gov | |
Description | Data deposited in or computed by PubChem | |
InChI |
InChI=1S/C11H11N3O/c1-15-9-4-2-3-8(5-9)10-6-11(12)14-7-13-10/h2-7H,1H3,(H2,12,13,14) | |
Source | PubChem | |
URL | https://pubchem.ncbi.nlm.nih.gov | |
Description | Data deposited in or computed by PubChem | |
InChI Key |
TUABPDBZFQJDHM-UHFFFAOYSA-N | |
Source | PubChem | |
URL | https://pubchem.ncbi.nlm.nih.gov | |
Description | Data deposited in or computed by PubChem | |
Canonical SMILES |
COC1=CC=CC(=C1)C2=CC(=NC=N2)N | |
Source | PubChem | |
URL | https://pubchem.ncbi.nlm.nih.gov | |
Description | Data deposited in or computed by PubChem | |
Molecular Formula |
C11H11N3O | |
Source | PubChem | |
URL | https://pubchem.ncbi.nlm.nih.gov | |
Description | Data deposited in or computed by PubChem | |
Molecular Weight |
201.22 g/mol | |
Source | PubChem | |
URL | https://pubchem.ncbi.nlm.nih.gov | |
Description | Data deposited in or computed by PubChem | |
Synthesis routes and methods
Procedure details
Retrosynthesis Analysis
AI-Powered Synthesis Planning: Our tool employs the Template_relevance Pistachio, Template_relevance Bkms_metabolic, Template_relevance Pistachio_ringbreaker, Template_relevance Reaxys, Template_relevance Reaxys_biocatalysis model, leveraging a vast database of chemical reactions to predict feasible synthetic routes.
One-Step Synthesis Focus: Specifically designed for one-step synthesis, it provides concise and direct routes for your target compounds, streamlining the synthesis process.
Accurate Predictions: Utilizing the extensive PISTACHIO, BKMS_METABOLIC, PISTACHIO_RINGBREAKER, REAXYS, REAXYS_BIOCATALYSIS database, our tool offers high-accuracy predictions, reflecting the latest in chemical research and data.
Strategy Settings
Precursor scoring | Relevance Heuristic |
---|---|
Min. plausibility | 0.01 |
Model | Template_relevance |
Template Set | Pistachio/Bkms_metabolic/Pistachio_ringbreaker/Reaxys/Reaxys_biocatalysis |
Top-N result to add to graph | 6 |
Feasible Synthetic Routes
Disclaimer and Information on In-Vitro Research Products
Please be aware that all articles and product information presented on BenchChem are intended solely for informational purposes. The products available for purchase on BenchChem are specifically designed for in-vitro studies, which are conducted outside of living organisms. In-vitro studies, derived from the Latin term "in glass," involve experiments performed in controlled laboratory settings using cells or tissues. It is important to note that these products are not categorized as medicines or drugs, and they have not received approval from the FDA for the prevention, treatment, or cure of any medical condition, ailment, or disease. We must emphasize that any form of bodily introduction of these products into humans or animals is strictly prohibited by law. It is essential to adhere to these guidelines to ensure compliance with legal and ethical standards in research and experimentation.