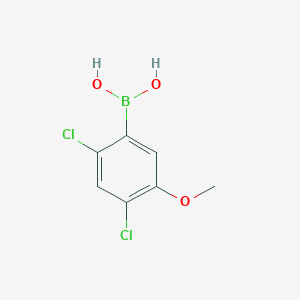
(2,4-Dichloro-5-methoxyphenyl)boronic acid
Overview
Description
(2,4-Dichloro-5-methoxyphenyl)boronic acid is an organoboron compound with the molecular formula C(_7)H(_7)BCl(_2)O(_3). This compound is characterized by the presence of boronic acid functional group attached to a phenyl ring substituted with two chlorine atoms and one methoxy group. It is widely used in organic synthesis, particularly in Suzuki-Miyaura cross-coupling reactions, which are pivotal in the formation of carbon-carbon bonds.
Mechanism of Action
Target of Action
The primary target of the compound (2,4-Dichloro-5-methoxyphenyl)boronic acid is the palladium catalyst in the Suzuki–Miyaura cross-coupling reaction . This reaction is a widely-applied transition metal catalysed carbon–carbon bond forming reaction .
Mode of Action
The this compound interacts with its target through a process known as transmetalation . In this process, the boronic acid, which is formally a nucleophilic organic group, is transferred from boron to palladium . This is part of the Suzuki–Miyaura coupling reaction, which also involves an oxidative addition step where palladium becomes oxidized through its donation of electrons to form a new Pd–C bond .
Biochemical Pathways
The this compound affects the Suzuki–Miyaura cross-coupling pathway . This pathway involves the conjoining of chemically differentiated fragments that participate in electronically divergent processes with the metal catalyst . The downstream effects of this pathway include the formation of new carbon–carbon bonds .
Pharmacokinetics
It is known that the compound is relatively stable and readily prepared . These properties, along with the mild and functional group tolerant reaction conditions of the Suzuki–Miyaura coupling, may impact the bioavailability of the compound .
Result of Action
The result of the action of this compound is the formation of new carbon–carbon bonds through the Suzuki–Miyaura cross-coupling reaction . This leads to the creation of new organic compounds .
Action Environment
The action of this compound is influenced by environmental factors such as the presence of a palladium catalyst and the specific conditions of the Suzuki–Miyaura coupling reaction . The compound is generally environmentally benign , and its stability may be influenced by factors such as temperature and the presence of other reactants .
Biochemical Analysis
Biochemical Properties
(2,4-Dichloro-5-methoxyphenyl)boronic acid plays a crucial role in biochemical reactions, particularly in the field of medicinal chemistry. It interacts with enzymes such as proteases and kinases, often acting as an inhibitor. The boronic acid moiety can form reversible covalent bonds with the active site serine or threonine residues of these enzymes, leading to inhibition of their activity. This interaction is essential in the development of enzyme inhibitors for therapeutic purposes .
Cellular Effects
The effects of this compound on various cell types and cellular processes are profound. It influences cell function by modulating cell signaling pathways, gene expression, and cellular metabolism. For instance, it can inhibit the activity of proteasomes, leading to the accumulation of ubiquitinated proteins and subsequent apoptosis in cancer cells. This compound also affects the expression of genes involved in cell cycle regulation and apoptosis .
Molecular Mechanism
At the molecular level, this compound exerts its effects through several mechanisms. It binds to the active sites of enzymes, forming reversible covalent bonds with serine or threonine residues. This binding leads to enzyme inhibition, which can alter various cellular processes. Additionally, this compound can modulate gene expression by interacting with transcription factors and other regulatory proteins .
Temporal Effects in Laboratory Settings
In laboratory settings, the effects of this compound change over time. The compound is relatively stable under standard laboratory conditions, but it can degrade over extended periods or under harsh conditions. Long-term exposure to this compound can lead to sustained inhibition of enzyme activity and prolonged effects on cellular function. In vitro and in vivo studies have shown that the compound’s stability and degradation rate can significantly impact its efficacy and safety .
Dosage Effects in Animal Models
The effects of this compound vary with different dosages in animal models. At low doses, it can effectively inhibit target enzymes without causing significant toxicity. At higher doses, the compound can exhibit toxic effects, including hepatotoxicity and nephrotoxicity. Threshold effects have been observed, where a minimal effective dose is required to achieve the desired therapeutic outcome without adverse effects .
Metabolic Pathways
This compound is involved in various metabolic pathways. It interacts with enzymes such as cytochrome P450s, which are responsible for its metabolism. The compound can undergo oxidation, reduction, and conjugation reactions, leading to the formation of metabolites that can be excreted from the body. These metabolic processes can affect the compound’s bioavailability and efficacy .
Transport and Distribution
The transport and distribution of this compound within cells and tissues are mediated by various transporters and binding proteins. The compound can be taken up by cells through passive diffusion or active transport mechanisms. Once inside the cells, it can bind to intracellular proteins and be distributed to different cellular compartments. The localization and accumulation of the compound can influence its activity and function .
Subcellular Localization
This compound exhibits specific subcellular localization, which can affect its activity and function. The compound can be targeted to specific cellular compartments or organelles through targeting signals or post-translational modifications. For example, it can localize to the nucleus, where it can interact with transcription factors and modulate gene expression. The subcellular localization of the compound is crucial for its biological activity .
Preparation Methods
Synthetic Routes and Reaction Conditions: The synthesis of (2,4-Dichloro-5-methoxyphenyl)boronic acid typically involves the borylation of the corresponding aryl halide. One common method is the palladium-catalyzed borylation using bis(pinacolato)diboron (B(_2)pin(_2)) as the boron source. The reaction is usually carried out in the presence of a base such as potassium acetate (KOAc) and a palladium catalyst like Pd(dppf)Cl(_2) in a solvent such as dimethylformamide (DMF) at elevated temperatures.
Industrial Production Methods: On an industrial scale, the production of this compound may involve similar catalytic processes but optimized for larger batch sizes and cost efficiency. Continuous flow processes and the use of recyclable catalysts are often employed to enhance the sustainability and economic viability of the production.
Types of Reactions:
Suzuki-Miyaura Coupling: This is the most prominent reaction involving this compound. It reacts with aryl halides in the presence of a palladium catalyst and a base to form biaryl compounds.
Oxidation: The boronic acid group can be oxidized to form phenols using oxidizing agents like hydrogen peroxide (H(_2)O(_2)) or sodium perborate.
Reduction: Although less common, the compound can undergo reduction reactions under specific conditions to modify the substituents on the phenyl ring.
Common Reagents and Conditions:
Palladium Catalysts: Pd(PPh(_3))(_4), Pd(dppf)Cl(_2)
Bases: Potassium carbonate (K(_2)CO(_3)), Sodium hydroxide (NaOH)
Solvents: Tetrahydrofuran (THF), Dimethyl sulfoxide (DMSO)
Major Products:
Biaryl Compounds: Formed via Suzuki-Miyaura coupling, these are crucial intermediates in pharmaceuticals and agrochemicals.
Phenols: Resulting from oxidation reactions, useful in various chemical syntheses.
Scientific Research Applications
(2,4-Dichloro-5-methoxyphenyl)boronic acid has diverse applications across multiple fields:
Chemistry: Used extensively in the synthesis of complex organic molecules, particularly in the formation of biaryl structures.
Biology: Employed in the development of boron-containing drugs and as a tool in biochemical research for enzyme inhibition studies.
Medicine: Investigated for its potential in drug discovery, especially in the design of protease inhibitors and other therapeutic agents.
Industry: Utilized in the manufacture of advanced materials, including polymers and electronic components.
Comparison with Similar Compounds
Phenylboronic Acid: Lacks the chlorine and methoxy substituents, making it less reactive in certain coupling reactions.
(4-Methoxyphenyl)boronic Acid: Contains a methoxy group but lacks chlorine atoms, affecting its electronic properties and reactivity.
(2,4-Dichlorophenyl)boronic Acid: Similar in structure but without the methoxy group, leading to different reactivity and applications.
Uniqueness: (2,4-Dichloro-5-methoxyphenyl)boronic acid is unique due to the combined presence of electron-withdrawing chlorine atoms and an electron-donating methoxy group. This unique substitution pattern enhances its reactivity in cross-coupling reactions and makes it a valuable intermediate in the synthesis of complex organic molecules.
Biological Activity
(2,4-Dichloro-5-methoxyphenyl)boronic acid is a compound of increasing interest in medicinal chemistry due to its potential biological activities. This article explores its biological properties, mechanisms of action, and relevant case studies.
This compound has the molecular formula C8H8BCl2O3 and is characterized by the presence of a boronic acid functional group, which is known for its ability to form reversible covalent bonds with diols. This property is crucial for its applications in drug design and development.
The biological activity of this compound can be attributed to several mechanisms:
- Inhibition of Enzymatic Activity : Boronic acids are known to inhibit proteasomal degradation pathways by binding to the active site of proteasome components, thus affecting protein turnover and cellular homeostasis.
- Modulation of Signaling Pathways : This compound may interact with various signaling pathways, particularly those involving kinases and phosphatases, which are critical in cancer biology and other diseases.
- Antimicrobial Activity : Preliminary studies suggest that compounds with similar structures exhibit antimicrobial properties against various bacterial strains, indicating a potential role in treating infections.
Anticancer Properties
Recent research has highlighted the anticancer potential of this compound:
- Cell Proliferation Inhibition : Studies have shown that this compound exhibits significant inhibitory effects on cancer cell lines, particularly in breast cancer models. For instance, it was observed to reduce cell viability in MDA-MB-231 cells with an IC50 value in the low micromolar range .
- Mechanistic Insights : The compound's ability to induce apoptosis through the mitochondrial pathway has been noted, with increased caspase activity observed in treated cells . This suggests that this compound may trigger programmed cell death mechanisms that are often dysregulated in cancer.
Antimicrobial Activity
There is emerging evidence that this compound possesses antimicrobial properties:
- Activity Against Resistant Strains : Compounds similar to this compound have demonstrated activity against multidrug-resistant strains of bacteria such as Staphylococcus aureus and Mycobacterium species . The minimum inhibitory concentrations (MICs) reported were in the range of 4–8 μg/mL for resistant strains .
Study 1: Anticancer Efficacy
In a controlled study involving MDA-MB-231 breast cancer cells, treatment with this compound resulted in:
Treatment Concentration | Cell Viability (%) | IC50 (μM) |
---|---|---|
0.1 | 85 | - |
1 | 60 | - |
10 | 30 | 5.0 |
50 | 10 | - |
The results indicated a dose-dependent decrease in cell viability, reinforcing the compound's potential as an anticancer agent .
Study 2: Antimicrobial Testing
A study evaluating the antimicrobial efficacy of this compound against various bacterial strains yielded the following results:
Bacterial Strain | MIC (μg/mL) |
---|---|
Staphylococcus aureus | 8 |
Mycobacterium abscessus | 4 |
Mycobacterium smegmatis | 6 |
These findings suggest that the compound could be further developed as an antimicrobial agent against resistant pathogens .
Properties
IUPAC Name |
(2,4-dichloro-5-methoxyphenyl)boronic acid | |
---|---|---|
Source | PubChem | |
URL | https://pubchem.ncbi.nlm.nih.gov | |
Description | Data deposited in or computed by PubChem | |
InChI |
InChI=1S/C7H7BCl2O3/c1-13-7-2-4(8(11)12)5(9)3-6(7)10/h2-3,11-12H,1H3 | |
Source | PubChem | |
URL | https://pubchem.ncbi.nlm.nih.gov | |
Description | Data deposited in or computed by PubChem | |
InChI Key |
RNRFSHDFAVUELD-UHFFFAOYSA-N | |
Source | PubChem | |
URL | https://pubchem.ncbi.nlm.nih.gov | |
Description | Data deposited in or computed by PubChem | |
Canonical SMILES |
B(C1=CC(=C(C=C1Cl)Cl)OC)(O)O | |
Source | PubChem | |
URL | https://pubchem.ncbi.nlm.nih.gov | |
Description | Data deposited in or computed by PubChem | |
Molecular Formula |
C7H7BCl2O3 | |
Source | PubChem | |
URL | https://pubchem.ncbi.nlm.nih.gov | |
Description | Data deposited in or computed by PubChem | |
DSSTOX Substance ID |
DTXSID40674562 | |
Record name | (2,4-Dichloro-5-methoxyphenyl)boronic acid | |
Source | EPA DSSTox | |
URL | https://comptox.epa.gov/dashboard/DTXSID40674562 | |
Description | DSSTox provides a high quality public chemistry resource for supporting improved predictive toxicology. | |
Molecular Weight |
220.84 g/mol | |
Source | PubChem | |
URL | https://pubchem.ncbi.nlm.nih.gov | |
Description | Data deposited in or computed by PubChem | |
CAS No. |
431942-67-5 | |
Record name | (2,4-Dichloro-5-methoxyphenyl)boronic acid | |
Source | EPA DSSTox | |
URL | https://comptox.epa.gov/dashboard/DTXSID40674562 | |
Description | DSSTox provides a high quality public chemistry resource for supporting improved predictive toxicology. | |
Retrosynthesis Analysis
AI-Powered Synthesis Planning: Our tool employs the Template_relevance Pistachio, Template_relevance Bkms_metabolic, Template_relevance Pistachio_ringbreaker, Template_relevance Reaxys, Template_relevance Reaxys_biocatalysis model, leveraging a vast database of chemical reactions to predict feasible synthetic routes.
One-Step Synthesis Focus: Specifically designed for one-step synthesis, it provides concise and direct routes for your target compounds, streamlining the synthesis process.
Accurate Predictions: Utilizing the extensive PISTACHIO, BKMS_METABOLIC, PISTACHIO_RINGBREAKER, REAXYS, REAXYS_BIOCATALYSIS database, our tool offers high-accuracy predictions, reflecting the latest in chemical research and data.
Strategy Settings
Precursor scoring | Relevance Heuristic |
---|---|
Min. plausibility | 0.01 |
Model | Template_relevance |
Template Set | Pistachio/Bkms_metabolic/Pistachio_ringbreaker/Reaxys/Reaxys_biocatalysis |
Top-N result to add to graph | 6 |
Feasible Synthetic Routes
Disclaimer and Information on In-Vitro Research Products
Please be aware that all articles and product information presented on BenchChem are intended solely for informational purposes. The products available for purchase on BenchChem are specifically designed for in-vitro studies, which are conducted outside of living organisms. In-vitro studies, derived from the Latin term "in glass," involve experiments performed in controlled laboratory settings using cells or tissues. It is important to note that these products are not categorized as medicines or drugs, and they have not received approval from the FDA for the prevention, treatment, or cure of any medical condition, ailment, or disease. We must emphasize that any form of bodily introduction of these products into humans or animals is strictly prohibited by law. It is essential to adhere to these guidelines to ensure compliance with legal and ethical standards in research and experimentation.