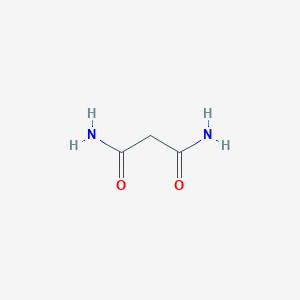
Malonamide
Overview
Description
Malonamide is an organic compound with the formula ( \mathrm{CH_2(CONH_2)_2} ). It is a derivative of malonic acid where both carboxyl groups are converted to amide groups.
Mechanism of Action
Target of Action
Malonamide is primarily used as an extractant in the separation of actinide (III) and lanthanide (III) ions from acidic media . It is also being explored as a potential antibacterial agent, particularly against Methicillin-resistant Staphylococcus aureus (MRSA) .
Mode of Action
It is known to interact with its targets through weak interactions that drive extractant assembly . In the case of its antibacterial activity, it is believed to potentiate the action of classic antimicrobials .
Biochemical Pathways
It is known that this compound’s mechanism of water extraction relies on the formation of 1:1 water-extractant adducts at low concentrations .
Pharmacokinetics
The synthesis of this compound derivatives has been reported, which could potentially influence its pharmacokinetic properties .
Result of Action
The primary result of this compound’s action is the extraction of certain ions from acidic media, which is useful in nuclear reprocessing . As an antibacterial agent, it has been shown to increase bacterial killing and biofilm eradication .
Action Environment
The efficacy and stability of this compound can be influenced by various environmental factors. For instance, the concentration of the extractant can affect the mechanism of water extraction
Biochemical Analysis
Biochemical Properties
Malonamide interacts with various biomolecules in biochemical reactions. In the context of antibacterial activity, this compound derivatives have been found to increase bacterial killing and biofilm eradication .
Cellular Effects
The effects of this compound on cells are primarily related to its antibacterial properties. It has been shown to increase bacterial killing and biofilm eradication, suggesting that it may influence cell function by disrupting bacterial cell signaling pathways, gene expression, and cellular metabolism .
Molecular Mechanism
Its antibacterial effects suggest that it may interact with biomolecules in bacteria, potentially inhibiting enzymes or altering gene expression to exert its effects .
Preparation Methods
Synthetic Routes and Reaction Conditions: Malonamide can be synthesized through several methods. One common approach involves the reaction of malonic acid with ammonia or amines. Another method includes the use of isocyanide-based multi-component reactions, which involve the condensation of isocyanide, Meldrum’s acid, arylidene malononitrile, and two amine molecules in dichloromethane at ambient temperature .
Industrial Production Methods: In industrial settings, this compound derivatives are often produced using multi-component reactions due to their efficiency and high atom economy. These reactions reduce the number of synthetic steps, solvent use, and energy consumption, making them cost-effective and environmentally friendly .
Chemical Reactions Analysis
Types of Reactions: Malonamide undergoes various chemical reactions, including:
Oxidation: this compound can be oxidized to produce malonic acid or its derivatives.
Reduction: Reduction of this compound can yield primary amines.
Substitution: this compound can participate in nucleophilic substitution reactions, where the amide groups can be replaced by other functional groups.
Common Reagents and Conditions:
Oxidation: Common oxidizing agents include potassium permanganate and hydrogen peroxide.
Reduction: Reducing agents such as lithium aluminum hydride or sodium borohydride are typically used.
Substitution: Nucleophiles like alkoxides or halides can be used under basic or acidic conditions.
Major Products:
Oxidation: Malonic acid or its esters.
Reduction: Primary amines.
Substitution: Various substituted this compound derivatives depending on the nucleophile used.
Scientific Research Applications
Malonamide and its derivatives have a wide range of applications in scientific research:
Chemistry: Used as building blocks in organic synthesis and as ligands in coordination chemistry.
Comparison with Similar Compounds
Malonamide can be compared with other similar compounds such as:
Malonic Acid: The parent compound of this compound, used primarily in organic synthesis.
Succinimide: Another amide derivative with applications in pharmaceuticals and as a precursor in organic synthesis.
Glutaramide: Similar in structure but with a longer carbon chain, used in polymer chemistry and as a pharmaceutical intermediate.
Uniqueness: this compound’s unique properties, such as its ability to form stable complexes with metal ions and its diverse biological activities, make it distinct from other similar compounds .
Properties
IUPAC Name |
propanediamide | |
---|---|---|
Source | PubChem | |
URL | https://pubchem.ncbi.nlm.nih.gov | |
Description | Data deposited in or computed by PubChem | |
InChI |
InChI=1S/C3H6N2O2/c4-2(6)1-3(5)7/h1H2,(H2,4,6)(H2,5,7) | |
Source | PubChem | |
URL | https://pubchem.ncbi.nlm.nih.gov | |
Description | Data deposited in or computed by PubChem | |
InChI Key |
WRIRWRKPLXCTFD-UHFFFAOYSA-N | |
Source | PubChem | |
URL | https://pubchem.ncbi.nlm.nih.gov | |
Description | Data deposited in or computed by PubChem | |
Canonical SMILES |
C(C(=O)N)C(=O)N | |
Source | PubChem | |
URL | https://pubchem.ncbi.nlm.nih.gov | |
Description | Data deposited in or computed by PubChem | |
Molecular Formula |
C3H6N2O2 | |
Source | PubChem | |
URL | https://pubchem.ncbi.nlm.nih.gov | |
Description | Data deposited in or computed by PubChem | |
DSSTOX Substance ID |
DTXSID1040116 | |
Record name | Malonamide | |
Source | EPA DSSTox | |
URL | https://comptox.epa.gov/dashboard/DTXSID1040116 | |
Description | DSSTox provides a high quality public chemistry resource for supporting improved predictive toxicology. | |
Molecular Weight |
102.09 g/mol | |
Source | PubChem | |
URL | https://pubchem.ncbi.nlm.nih.gov | |
Description | Data deposited in or computed by PubChem | |
Physical Description |
White crystalline solid; [Sigma-Aldrich MSDS] | |
Record name | Malonamide | |
Source | Haz-Map, Information on Hazardous Chemicals and Occupational Diseases | |
URL | https://haz-map.com/Agents/20726 | |
Description | Haz-Map® is an occupational health database designed for health and safety professionals and for consumers seeking information about the adverse effects of workplace exposures to chemical and biological agents. | |
Explanation | Copyright (c) 2022 Haz-Map(R). All rights reserved. Unless otherwise indicated, all materials from Haz-Map are copyrighted by Haz-Map(R). No part of these materials, either text or image may be used for any purpose other than for personal use. Therefore, reproduction, modification, storage in a retrieval system or retransmission, in any form or by any means, electronic, mechanical or otherwise, for reasons other than personal use, is strictly prohibited without prior written permission. | |
CAS No. |
108-13-4 | |
Record name | Malonamide | |
Source | CAS Common Chemistry | |
URL | https://commonchemistry.cas.org/detail?cas_rn=108-13-4 | |
Description | CAS Common Chemistry is an open community resource for accessing chemical information. Nearly 500,000 chemical substances from CAS REGISTRY cover areas of community interest, including common and frequently regulated chemicals, and those relevant to high school and undergraduate chemistry classes. This chemical information, curated by our expert scientists, is provided in alignment with our mission as a division of the American Chemical Society. | |
Explanation | The data from CAS Common Chemistry is provided under a CC-BY-NC 4.0 license, unless otherwise stated. | |
Record name | Malonamide | |
Source | ChemIDplus | |
URL | https://pubchem.ncbi.nlm.nih.gov/substance/?source=chemidplus&sourceid=0000108134 | |
Description | ChemIDplus is a free, web search system that provides access to the structure and nomenclature authority files used for the identification of chemical substances cited in National Library of Medicine (NLM) databases, including the TOXNET system. | |
Record name | MALONAMIDE | |
Source | DTP/NCI | |
URL | https://dtp.cancer.gov/dtpstandard/servlet/dwindex?searchtype=NSC&outputformat=html&searchlist=2134 | |
Description | The NCI Development Therapeutics Program (DTP) provides services and resources to the academic and private-sector research communities worldwide to facilitate the discovery and development of new cancer therapeutic agents. | |
Explanation | Unless otherwise indicated, all text within NCI products is free of copyright and may be reused without our permission. Credit the National Cancer Institute as the source. | |
Record name | Propanediamide | |
Source | EPA Chemicals under the TSCA | |
URL | https://www.epa.gov/chemicals-under-tsca | |
Description | EPA Chemicals under the Toxic Substances Control Act (TSCA) collection contains information on chemicals and their regulations under TSCA, including non-confidential content from the TSCA Chemical Substance Inventory and Chemical Data Reporting. | |
Record name | Malonamide | |
Source | EPA DSSTox | |
URL | https://comptox.epa.gov/dashboard/DTXSID1040116 | |
Description | DSSTox provides a high quality public chemistry resource for supporting improved predictive toxicology. | |
Record name | Malonamide | |
Source | European Chemicals Agency (ECHA) | |
URL | https://echa.europa.eu/substance-information/-/substanceinfo/100.003.231 | |
Description | The European Chemicals Agency (ECHA) is an agency of the European Union which is the driving force among regulatory authorities in implementing the EU's groundbreaking chemicals legislation for the benefit of human health and the environment as well as for innovation and competitiveness. | |
Explanation | Use of the information, documents and data from the ECHA website is subject to the terms and conditions of this Legal Notice, and subject to other binding limitations provided for under applicable law, the information, documents and data made available on the ECHA website may be reproduced, distributed and/or used, totally or in part, for non-commercial purposes provided that ECHA is acknowledged as the source: "Source: European Chemicals Agency, http://echa.europa.eu/". Such acknowledgement must be included in each copy of the material. ECHA permits and encourages organisations and individuals to create links to the ECHA website under the following cumulative conditions: Links can only be made to webpages that provide a link to the Legal Notice page. | |
Record name | MALONAMIDE | |
Source | FDA Global Substance Registration System (GSRS) | |
URL | https://gsrs.ncats.nih.gov/ginas/app/beta/substances/QVQ8CNG255 | |
Description | The FDA Global Substance Registration System (GSRS) enables the efficient and accurate exchange of information on what substances are in regulated products. Instead of relying on names, which vary across regulatory domains, countries, and regions, the GSRS knowledge base makes it possible for substances to be defined by standardized, scientific descriptions. | |
Explanation | Unless otherwise noted, the contents of the FDA website (www.fda.gov), both text and graphics, are not copyrighted. They are in the public domain and may be republished, reprinted and otherwise used freely by anyone without the need to obtain permission from FDA. Credit to the U.S. Food and Drug Administration as the source is appreciated but not required. | |
Retrosynthesis Analysis
AI-Powered Synthesis Planning: Our tool employs the Template_relevance Pistachio, Template_relevance Bkms_metabolic, Template_relevance Pistachio_ringbreaker, Template_relevance Reaxys, Template_relevance Reaxys_biocatalysis model, leveraging a vast database of chemical reactions to predict feasible synthetic routes.
One-Step Synthesis Focus: Specifically designed for one-step synthesis, it provides concise and direct routes for your target compounds, streamlining the synthesis process.
Accurate Predictions: Utilizing the extensive PISTACHIO, BKMS_METABOLIC, PISTACHIO_RINGBREAKER, REAXYS, REAXYS_BIOCATALYSIS database, our tool offers high-accuracy predictions, reflecting the latest in chemical research and data.
Strategy Settings
Precursor scoring | Relevance Heuristic |
---|---|
Min. plausibility | 0.01 |
Model | Template_relevance |
Template Set | Pistachio/Bkms_metabolic/Pistachio_ringbreaker/Reaxys/Reaxys_biocatalysis |
Top-N result to add to graph | 6 |
Feasible Synthetic Routes
Disclaimer and Information on In-Vitro Research Products
Please be aware that all articles and product information presented on BenchChem are intended solely for informational purposes. The products available for purchase on BenchChem are specifically designed for in-vitro studies, which are conducted outside of living organisms. In-vitro studies, derived from the Latin term "in glass," involve experiments performed in controlled laboratory settings using cells or tissues. It is important to note that these products are not categorized as medicines or drugs, and they have not received approval from the FDA for the prevention, treatment, or cure of any medical condition, ailment, or disease. We must emphasize that any form of bodily introduction of these products into humans or animals is strictly prohibited by law. It is essential to adhere to these guidelines to ensure compliance with legal and ethical standards in research and experimentation.