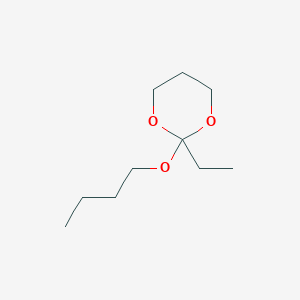
2-Butoxy-2-ethyl-1,3-dioxane
- Click on QUICK INQUIRY to receive a quote from our team of experts.
- With the quality product at a COMPETITIVE price, you can focus more on your research.
Overview
Description
2-Butoxy-2-ethyl-1,3-dioxane is an organic compound belonging to the class of dioxanes. Dioxanes are a group of heterocyclic organic compounds characterized by a six-membered ring containing two oxygen atoms. This particular compound is notable for its unique structure, which includes a butoxy and an ethyl group attached to the dioxane ring.
Preparation Methods
Synthetic Routes and Reaction Conditions
2-Butoxy-2-ethyl-1,3-dioxane can be synthesized through the acetalization of carbonyl compounds with 1,3-propanediol or 1,2-ethanediol in the presence of a Brönsted or Lewis acid catalyst . The reaction typically involves refluxing toluene with toluenesulfonic acid as the catalyst, allowing for the continuous removal of water from the reaction mixture using a Dean-Stark apparatus . Other methods include the use of trialkyl orthoformate and a catalytic amount of tetrabutylammonium tribromide in absolute alcohol .
Industrial Production Methods
Industrial production of this compound follows similar synthetic routes but on a larger scale. The use of efficient catalysts such as zirconium tetrachloride (ZrCl4) for acetalization and in situ transacetalization of carbonyl compounds under mild reaction conditions is common . The process ensures high yields and purity of the final product.
Chemical Reactions Analysis
Types of Reactions
2-Butoxy-2-ethyl-1,3-dioxane undergoes various chemical reactions, including:
Oxidation: The compound can be oxidized using agents such as potassium permanganate (KMnO4) or osmium tetroxide (OsO4).
Substitution: The compound can undergo substitution reactions with nucleophiles such as organolithium (RLi) or Grignard reagents (RMgX).
Common Reagents and Conditions
Oxidation: KMnO4, OsO4, CrO3/Py, RCOOOH, I2, Br2, Cl2, MnO2/CH2Cl2.
Reduction: H2/Ni, H2/Rh, Zn/HCl, Na/NH3, LiAlH4, NaBH4.
Substitution: RLi, RMgX, RCuLi, NH3, RNH2, NaOCH3.
Major Products Formed
The major products formed from these reactions depend on the specific reagents and conditions used. For example, oxidation may yield carboxylic acids or ketones, while reduction may produce alcohols or alkanes .
Scientific Research Applications
2-Butoxy-2-ethyl-1,3-dioxane has several applications in scientific research:
Chemistry: It is used as a solvent and reagent in various organic synthesis reactions.
Biology: The compound is studied for its potential biological activity and interactions with biomolecules.
Medicine: Research is ongoing to explore its potential therapeutic applications.
Industry: It is used in the production of polymers, resins, and other industrial chemicals.
Mechanism of Action
The mechanism by which 2-Butoxy-2-ethyl-1,3-dioxane exerts its effects involves its interaction with molecular targets and pathways. The compound can act as a nucleophile or electrophile in various chemical reactions, facilitating the formation or cleavage of chemical bonds . Its unique structure allows it to participate in a wide range of chemical processes, making it a versatile compound in both research and industrial applications.
Comparison with Similar Compounds
Similar Compounds
1,3-Dioxane: A simpler dioxane compound with similar chemical properties.
1,3-Dioxolane: Another related compound with a five-membered ring structure.
2,5-Diphenyl-1,3-oxazoline: A compound with a similar heterocyclic structure but different functional groups.
Uniqueness
2-Butoxy-2-ethyl-1,3-dioxane is unique due to its specific functional groups (butoxy and ethyl) attached to the dioxane ring. These groups confer distinct chemical properties and reactivity, making it suitable for specialized applications in organic synthesis and industrial processes .
Properties
CAS No. |
923035-52-3 |
---|---|
Molecular Formula |
C10H20O3 |
Molecular Weight |
188.26 g/mol |
IUPAC Name |
2-butoxy-2-ethyl-1,3-dioxane |
InChI |
InChI=1S/C10H20O3/c1-3-5-7-11-10(4-2)12-8-6-9-13-10/h3-9H2,1-2H3 |
InChI Key |
OFAWFLSITGZQNI-UHFFFAOYSA-N |
Canonical SMILES |
CCCCOC1(OCCCO1)CC |
Origin of Product |
United States |
Disclaimer and Information on In-Vitro Research Products
Please be aware that all articles and product information presented on BenchChem are intended solely for informational purposes. The products available for purchase on BenchChem are specifically designed for in-vitro studies, which are conducted outside of living organisms. In-vitro studies, derived from the Latin term "in glass," involve experiments performed in controlled laboratory settings using cells or tissues. It is important to note that these products are not categorized as medicines or drugs, and they have not received approval from the FDA for the prevention, treatment, or cure of any medical condition, ailment, or disease. We must emphasize that any form of bodily introduction of these products into humans or animals is strictly prohibited by law. It is essential to adhere to these guidelines to ensure compliance with legal and ethical standards in research and experimentation.