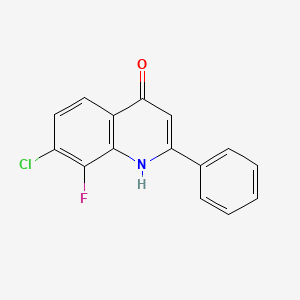
7-Chloro-8-fluoro-2-phenylquinolin-4(1H)-one
Overview
Description
7-Chloro-8-fluoro-2-phenylquinolin-4(1H)-one is a substituted quinolinone derivative characterized by a chlorine atom at position 7, a fluorine atom at position 8, and a phenyl group at position 2 of the quinoline ring. The quinolinone scaffold is known for its versatility in medicinal chemistry, often serving as a core structure for bioactive molecules.
Preparation Methods
Synthetic Routes and Reaction Conditions
Multi-step Synthesis from Readily Available Precursors
The most common approach involves constructing the quinoline nucleus followed by selective halogenation and functionalization:
Formation of the Quinoline Core:
The Skraup synthesis is frequently employed, which involves condensing aniline with glycerol in the presence of an oxidizing agent such as nitrobenzene, forming the quinoline skeleton. This classical method is adaptable for subsequent substitution steps.Introduction of Halogen Substituents:
Halogenation steps are performed post-core synthesis:- Chlorination : Achieved using thionyl chloride ($$\mathrm{SOCl_2}$$), which replaces hydroxyl groups or activates positions for substitution.
- Fluorination : Typically involves electrophilic fluorinating agents such as N-fluorobenzenesulfonimide (NFSI) or Selectfluor, which facilitate regioselective fluorine introduction at the desired position.
Phenyl Group Incorporation:
The phenyl moiety is introduced via Friedel-Crafts acylation using benzoyl chloride and aluminum chloride ($$\mathrm{AlCl_3}$$), followed by cyclization to form the quinoline ring system.
Specific Synthetic Pathway Example
Based on research data, a representative synthesis includes:
Step | Reaction | Reagents | Conditions | Purpose |
---|---|---|---|---|
1 | Quinoline core synthesis | Aniline + glycerol | Reflux, oxidant | Core formation |
2 | Chlorination | Thionyl chloride | Reflux | Chlorine substitution |
3 | Fluorination | NFSI or Selectfluor | Room temperature | Fluorine introduction |
4 | Phenyl addition | Benzoyl chloride + AlCl$$_3$$ | Reflux | Phenyl group attachment |
Research Findings and Method Optimization
Laboratory-Scale Synthesis
Research articles highlight the use of solvent-free Friedländer synthesis, which enhances yield and reduces environmental impact. For example, one study describes synthesizing quinoline derivatives via heating substituted anilines with ketones under polyphosphoric acid (PPA) catalysis, achieving high purity and yield (up to 60%).
Industrial-Scale Production
Industrial synthesis optimizes reaction conditions for large-scale manufacturing, often employing continuous flow reactors, which improve safety, efficiency, and control over halogenation steps. Purification techniques such as chromatography and recrystallization are employed to obtain high-purity compounds suitable for pharmaceutical applications.
Data Tables Summarizing Preparation Methods
Method | Starting Materials | Key Reagents | Reaction Conditions | Advantages | Limitations |
---|---|---|---|---|---|
Classical Multi-step | Aniline, glycerol, benzoyl chloride | $$\mathrm{SOCl2}$$, $$\mathrm{AlCl3}$$, NFSI | Reflux, room temp | Versatile, high yield | Time-consuming, multiple steps |
Solvent-free Friedländer | Substituted aniline, ketone | PPA | Heating at 130°C | Environmentally friendly, high efficiency | Requires precise temperature control |
Industrial Continuous Flow | Various precursors | Halogenating agents | Controlled flow reactions | Scalable, consistent quality | Equipment complexity |
Notable Research Findings and Innovations
Enhanced Synthesis via Solvent-Free Methods:
Recent research demonstrates that solvent-free Friedländer reactions using polyphosphoric acid significantly improve yield and reduce waste, aligning with green chemistry principles.Selective Halogenation Techniques:
Advances in electrophilic halogenation have enabled regioselective introduction of chlorine and fluorine, crucial for biological activity optimization.Catalyst Optimization:
Use of Lewis acids like $$\mathrm{AlCl3}$$ or $$\mathrm{FeCl3}$$ enhances phenyl group attachment efficiency, with reaction conditions fine-tuned for maximum selectivity.
Summary of Key Preparation Data
Technique | Yield (%) | Reaction Time | Reagents | Notes |
---|---|---|---|---|
Classical multi-step | 50-70 | 24-48 hours | Aniline, glycerol, halogenating agents | Widely used, adaptable |
Solvent-free Friedländer | 60-80 | 2-6 hours | Aniline derivatives, PPA | Eco-friendly, high yield |
Flow chemistry | 70-85 | Continuous | Precursors + halogenating agents | Scalable, consistent |
Chemical Reactions Analysis
Types of Reactions
7-Chloro-8-fluoro-2-phenylquinolin-4(1H)-one can undergo various chemical reactions, including:
Oxidation: The compound can be oxidized using oxidizing agents like potassium permanganate or chromium trioxide.
Reduction: Reduction can be achieved using reducing agents such as lithium aluminum hydride or sodium borohydride.
Substitution: The chloro and fluoro groups can be substituted with other functional groups through nucleophilic substitution reactions.
Common Reagents and Conditions
Oxidation: Potassium permanganate in acidic or basic medium.
Reduction: Lithium aluminum hydride in dry ether.
Substitution: Sodium methoxide in methanol for nucleophilic substitution.
Major Products Formed
Oxidation: Formation of quinoline N-oxide derivatives.
Reduction: Formation of reduced quinoline derivatives.
Substitution: Formation of substituted quinoline derivatives with various functional groups.
Scientific Research Applications
Medicinal Chemistry
7-Chloro-8-fluoro-2-phenylquinolin-4(1H)-one has shown promise in various therapeutic areas:
- Anticancer Activity : Research indicates that this compound exhibits significant antiproliferative effects against various cancer cell lines, including HL-60, Hep3B, and COLO 205. Mechanistic studies suggest that it disrupts microtubule assembly, leading to G2/M cell cycle arrest and apoptosis via intrinsic and extrinsic signaling pathways .
- Antimicrobial Properties : Quinoline derivatives are known for their antimicrobial activities, with studies highlighting the potential of this compound against various bacterial strains.
Pharmacological Studies
The compound is being investigated for its interactions with specific molecular targets:
- Enzyme Inhibition : It may inhibit key enzymes involved in cancer cell proliferation, making it a potential candidate for cancer therapy.
- Binding Affinity Studies : Interaction studies focus on its binding to enzymes and receptors, which is crucial for understanding its mechanism of action.
Material Science
In addition to its biological applications, this compound is also explored in material science for developing new chemical processes and materials due to its unique structural properties.
Anticancer Activity Assessment
A study evaluated various derivatives of quinolinone compounds against cancer cell lines. Notably, certain derivatives demonstrated high potency (IC50 < 1 μM) against selected cancer cells while sparing normal cells (IC50 > 50 μM) . This highlights the therapeutic potential of structurally related compounds like this compound.
Mechanistic Insights
Research has shown that compounds similar to this compound can induce apoptosis through various pathways, including microtubule disruption and activation of apoptotic proteins. These findings support the ongoing exploration of quinoline derivatives as "privileged structures" in drug development .
Mechanism of Action
The mechanism of action of 7-Chloro-8-fluoro-2-phenylquinolin-4(1H)-one involves its interaction with specific molecular targets. For example, it may inhibit certain enzymes or receptors, leading to its biological effects. The exact pathways and targets can vary depending on the specific application and context of use.
Comparison with Similar Compounds
Structural and Substitution Patterns
Key structural analogues differ in substituent type, position, and electronic effects:
Key Observations :
- Aromatic vs. Aliphatic Groups : The 2-phenyl group in the target compound may improve lipophilicity and π-π stacking interactions compared to aliphatic substituents like trifluoromethyl () or piperazine (), influencing bioavailability.
Physicochemical Properties
Critical properties such as density, pKa, and solubility vary with substituents:
Key Observations :
- Density : The trifluoromethyl-containing analogue () exhibits higher density (1.610 g/cm³) due to fluorine’s atomic mass, suggesting that halogen-rich derivatives may have increased molecular packing efficiency.
- Acidity : The pKa of 5.95 for the trifluoromethyl analogue () indicates moderate acidity, likely influenced by electron-withdrawing substituents stabilizing deprotonation.
Spectroscopic Characterization
NMR and mass spectrometry data highlight substituent-driven shifts:
- 1H-NMR :
- The target compound’s phenyl group (position 2) would produce aromatic proton signals near δ 7.2–7.8 ppm, distinct from trifluoromethyl (δ 3.7–4.2 ppm for adjacent protons) or piperazine (δ 2.1–3.5 ppm) substituents in analogues .
- The 8-fluoro substituent may deshield nearby protons, causing downfield shifts compared to methyl groups (e.g., δ 2.5 ppm for CH3 in ).
- Mass Spectrometry :
- The molecular ion peak (M⁺) for the target compound is expected near m/z 283.68, while the piperazine-containing analogue () shows M⁺ at m/z 393.14, reflecting its larger substituents.
Biological Activity
7-Chloro-8-fluoro-2-phenylquinolin-4(1H)-one is a compound belonging to the quinoline family, which has garnered attention for its diverse biological activities. This article delves into the biological activity of this compound, highlighting its potential therapeutic applications, mechanisms of action, and relevant research findings.
Overview of the Compound
This compound is characterized by its unique structure, which includes a quinoline core with chloro and fluoro substituents as well as a phenyl group. This structural configuration is believed to enhance its biological activity compared to other quinoline derivatives. Quinoline derivatives are known for their antimicrobial, anticancer, and anti-inflammatory properties, making them significant in medicinal chemistry.
The biological activity of this compound is primarily attributed to its interaction with various molecular targets:
- Enzyme Inhibition : The compound has been studied for its ability to inhibit specific enzymes involved in disease pathways. For instance, it may inhibit lactate dehydrogenase (LDH), which plays a crucial role in cancer metabolism .
- Antimicrobial Activity : Similar compounds in the quinoline family have demonstrated effectiveness against various pathogens, suggesting that this compound may also possess antimicrobial properties .
Anticancer Activity
Recent studies have highlighted the anticancer potential of this compound. In vitro assays have shown that this compound exhibits significant cytotoxicity against several cancer cell lines, including:
Cell Line | IC50 (µM) | Mechanism |
---|---|---|
HL-60 | < 1 | Induces apoptosis through intrinsic pathways |
Hep3B | < 1 | Disrupts microtubule assembly |
COLO 205 | < 1 | G2/M arrest and apoptosis induction |
These findings indicate that the compound can selectively target cancer cells while sparing normal cells, which is crucial for reducing side effects in therapeutic applications .
Antimicrobial Properties
The antimicrobial efficacy of this compound has been explored against various bacterial strains. Preliminary results suggest that it may inhibit bacterial growth effectively, although further studies are needed to establish its full spectrum of activity and mechanism .
Case Studies
- Case Study on Anticancer Activity : A study involving a series of quinoline derivatives demonstrated that modifications on the quinoline core significantly influenced their anticancer activity. The presence of both chloro and fluoro groups in this compound was found to enhance its potency against cancer cell lines compared to simpler analogs .
- Case Study on Enzyme Inhibition : Another study focused on the inhibition of LDH by various quinoline derivatives. It was found that compounds similar to this compound exhibited competitive inhibition, suggesting potential for use in metabolic disorders and cancer therapy .
Q & A
Q. Basic: What synthetic strategies are effective for preparing 7-chloro-8-fluoro-2-phenylquinolin-4(1H)-one?
Answer:
The synthesis typically involves cyclization of substituted aniline precursors or modification of preformed quinoline scaffolds. Key steps include:
- Cyclocondensation : Reacting substituted 2-aminobenzophenones with chloro/fluoro-substituted malonyl derivatives under acidic conditions (e.g., HCl or acetic acid) to form the quinolinone core .
- Halogenation : Introducing fluorine at the 8-position via electrophilic fluorination agents (e.g., Selectfluor®) or nucleophilic displacement of leaving groups (e.g., nitro or sulfonate) under basic conditions .
- Characterization : Confirming purity and structure using ¹H/¹³C NMR, HRMS, and IR spectroscopy .
Q. Basic: What analytical techniques are critical for confirming the structure of this compound?
Answer:
- ¹H/¹³C NMR : Assign signals for the quinolinone core (e.g., carbonyl at ~δ 175 ppm in ¹³C NMR) and substituents (e.g., phenyl protons at δ 7.2–7.8 ppm). Fluorine coupling patterns (e.g., ⁸J-F splitting) confirm the 8-fluoro position .
- HRMS : Validate molecular formula (C₁₅H₈ClFNO) with accurate mass measurement (e.g., observed [M+H]⁺ at m/z 284.0245) .
- X-ray crystallography : Resolve ambiguities in regiochemistry, especially for distinguishing chloro/fluoro positions .
Q. Advanced: How can reaction conditions be optimized to minimize byproducts during fluorination?
Answer:
Fluorination at the 8-position is prone to side reactions (e.g., over-fluorination or ring degradation). Optimization strategies include:
- Temperature control : Conduct fluorination at 0–25°C to reduce thermal decomposition .
- Catalyst screening : Test Lewis acids (e.g., MgCl₂) or phase-transfer catalysts to enhance selectivity .
- Solvent effects : Polar aprotic solvents (e.g., DMF or acetonitrile) improve reagent solubility and reaction homogeneity .
- In-situ monitoring : Use TLC or HPLC to track reaction progress and terminate before byproduct formation .
Q. Advanced: How do electronic effects of the 2-phenyl substituent influence the compound’s reactivity?
Answer:
The electron-rich phenyl group at position 2 stabilizes the quinolinone core via resonance, affecting:
- Acid-base properties : Increased electron density at the 4-keto group lowers pKa, enhancing solubility in basic media .
- Electrophilic substitution : Directs incoming electrophiles to the 5- or 7-position due to meta/para activation by the phenyl ring .
- Photostability : Conjugation with the phenyl group reduces UV-induced degradation, critical for photophysical studies .
Q. Advanced: How to resolve discrepancies in NMR data for derivatives of this compound?
Answer:
Contradictions in NMR assignments often arise from dynamic processes (e.g., keto-enol tautomerism) or solvent effects. Mitigation approaches:
- Variable-temperature NMR : Identify tautomeric shifts (e.g., coalescence temperatures for enol/keto forms) .
- Deuterated solvents : Compare spectra in DMSO-d₆ vs. CDCl₃ to assess hydrogen bonding interactions .
- 2D NMR (COSY, HSQC) : Resolve overlapping signals by correlating ¹H-¹H and ¹H-¹³C couplings .
Q. Advanced: What methodologies are effective for studying the compound’s biological activity?
Answer:
- In-silico docking : Screen against target proteins (e.g., kinases or microbial enzymes) using software like AutoDock to predict binding modes .
- Enzyme assays : Measure IC₅₀ values for enzyme inhibition (e.g., antimalarial activity against Plasmodium lactate dehydrogenase) .
- Cytotoxicity profiling : Use MTT assays on mammalian cell lines to assess selectivity and therapeutic index .
Q. Advanced: How to design a stability study for this compound under varying pH conditions?
Answer:
- Forced degradation : Expose the compound to acidic (HCl, pH 1–3), neutral (water, pH 7), and basic (NaOH, pH 10–12) conditions at 40–60°C for 24–72 hours .
- Analytical endpoints : Monitor degradation via HPLC-MS to identify hydrolytic (e.g., ring-opening) or oxidative byproducts .
- Kinetic modeling : Calculate degradation rate constants (k) and shelf-life predictions using Arrhenius equations .
Properties
IUPAC Name |
7-chloro-8-fluoro-2-phenyl-1H-quinolin-4-one | |
---|---|---|
Source | PubChem | |
URL | https://pubchem.ncbi.nlm.nih.gov | |
Description | Data deposited in or computed by PubChem | |
InChI |
InChI=1S/C15H9ClFNO/c16-11-7-6-10-13(19)8-12(18-15(10)14(11)17)9-4-2-1-3-5-9/h1-8H,(H,18,19) | |
Source | PubChem | |
URL | https://pubchem.ncbi.nlm.nih.gov | |
Description | Data deposited in or computed by PubChem | |
InChI Key |
HZUOLYGWXKPPNY-UHFFFAOYSA-N | |
Source | PubChem | |
URL | https://pubchem.ncbi.nlm.nih.gov | |
Description | Data deposited in or computed by PubChem | |
Canonical SMILES |
C1=CC=C(C=C1)C2=CC(=O)C3=C(N2)C(=C(C=C3)Cl)F | |
Source | PubChem | |
URL | https://pubchem.ncbi.nlm.nih.gov | |
Description | Data deposited in or computed by PubChem | |
Molecular Formula |
C15H9ClFNO | |
Source | PubChem | |
URL | https://pubchem.ncbi.nlm.nih.gov | |
Description | Data deposited in or computed by PubChem | |
DSSTOX Substance ID |
DTXSID90656277 | |
Record name | 7-Chloro-8-fluoro-2-phenylquinolin-4(1H)-one | |
Source | EPA DSSTox | |
URL | https://comptox.epa.gov/dashboard/DTXSID90656277 | |
Description | DSSTox provides a high quality public chemistry resource for supporting improved predictive toxicology. | |
Molecular Weight |
273.69 g/mol | |
Source | PubChem | |
URL | https://pubchem.ncbi.nlm.nih.gov | |
Description | Data deposited in or computed by PubChem | |
CAS No. |
867165-00-2 | |
Record name | 7-Chloro-8-fluoro-2-phenylquinolin-4(1H)-one | |
Source | EPA DSSTox | |
URL | https://comptox.epa.gov/dashboard/DTXSID90656277 | |
Description | DSSTox provides a high quality public chemistry resource for supporting improved predictive toxicology. | |
Synthesis routes and methods
Procedure details
Retrosynthesis Analysis
AI-Powered Synthesis Planning: Our tool employs the Template_relevance Pistachio, Template_relevance Bkms_metabolic, Template_relevance Pistachio_ringbreaker, Template_relevance Reaxys, Template_relevance Reaxys_biocatalysis model, leveraging a vast database of chemical reactions to predict feasible synthetic routes.
One-Step Synthesis Focus: Specifically designed for one-step synthesis, it provides concise and direct routes for your target compounds, streamlining the synthesis process.
Accurate Predictions: Utilizing the extensive PISTACHIO, BKMS_METABOLIC, PISTACHIO_RINGBREAKER, REAXYS, REAXYS_BIOCATALYSIS database, our tool offers high-accuracy predictions, reflecting the latest in chemical research and data.
Strategy Settings
Precursor scoring | Relevance Heuristic |
---|---|
Min. plausibility | 0.01 |
Model | Template_relevance |
Template Set | Pistachio/Bkms_metabolic/Pistachio_ringbreaker/Reaxys/Reaxys_biocatalysis |
Top-N result to add to graph | 6 |
Feasible Synthetic Routes
Disclaimer and Information on In-Vitro Research Products
Please be aware that all articles and product information presented on BenchChem are intended solely for informational purposes. The products available for purchase on BenchChem are specifically designed for in-vitro studies, which are conducted outside of living organisms. In-vitro studies, derived from the Latin term "in glass," involve experiments performed in controlled laboratory settings using cells or tissues. It is important to note that these products are not categorized as medicines or drugs, and they have not received approval from the FDA for the prevention, treatment, or cure of any medical condition, ailment, or disease. We must emphasize that any form of bodily introduction of these products into humans or animals is strictly prohibited by law. It is essential to adhere to these guidelines to ensure compliance with legal and ethical standards in research and experimentation.