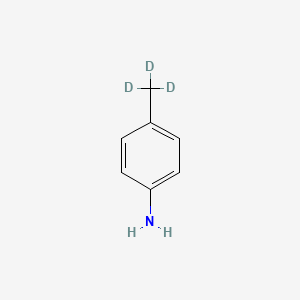
P-Toluidine-D3 (methyl-D3)
Overview
Description
P-Toluidine-D3 (methyl-D3) is a deuterated form of p-toluidine, where the methyl group is replaced with a deuterium-labeled methyl group. This compound is an isotopically labeled analog of p-toluidine, which is an aromatic amine. The chemical structure of p-toluidine-D3 (methyl-D3) consists of a benzene ring substituted with an amino group (NH2) and a deuterated methyl group (CD3) at the para position.
Preparation Methods
Synthetic Routes and Reaction Conditions
The synthesis of p-toluidine-D3 (methyl-D3) typically involves the deuteration of p-toluidine. One common method is the catalytic exchange of hydrogen atoms with deuterium using deuterium gas (D2) in the presence of a catalyst such as palladium on carbon (Pd/C). The reaction is carried out under mild conditions to ensure selective deuteration of the methyl group.
Industrial Production Methods
Industrial production of p-toluidine-D3 (methyl-D3) follows similar synthetic routes but on a larger scale. The process involves the use of high-pressure reactors and efficient catalysts to achieve high yields and purity. The deuterated compound is then purified using techniques such as distillation or crystallization.
Chemical Reactions Analysis
Types of Reactions
P-Toluidine-D3 (methyl-D3) undergoes various chemical reactions, including:
Oxidation: The compound can be oxidized to form p-toluidine derivatives such as 4,4’-dimethylazobenzene and 4,4’-dimethylazoxybenzene.
Reduction: Reduction reactions can convert p-toluidine-D3 (methyl-D3) to its corresponding amine derivatives.
Substitution: The amino group in p-toluidine-D3 (methyl-D3) can participate in electrophilic substitution reactions, leading to the formation of various substituted aromatic compounds.
Common Reagents and Conditions
Oxidation: Hydrogen peroxide (H2O2) in the presence of catalysts such as magnetite supported on nanocrystalline titanium silicalite-1 (M/NTS) zeolite.
Reduction: Sodium borohydride (NaBH4) or lithium aluminum hydride (LiAlH4) under mild conditions.
Substitution: Electrophilic reagents such as bromine (Br2) or nitronium ion (NO2+).
Major Products
Oxidation: 4,4’-dimethylazobenzene and 4,4’-dimethylazoxybenzene.
Reduction: Corresponding amine derivatives.
Substitution: Various substituted aromatic compounds depending on the electrophile used.
Scientific Research Applications
P-Toluidine-D3 (methyl-D3) is widely used in scientific research due to its isotopic labeling. Some of its applications include:
Chemistry: Used as a tracer in reaction mechanisms and kinetic studies to understand the behavior of p-toluidine in various chemical reactions.
Biology: Employed in metabolic studies to trace the pathways and interactions of p-toluidine in biological systems.
Medicine: Utilized in pharmacokinetic studies to investigate the absorption, distribution, metabolism, and excretion of p-toluidine derivatives.
Industry: Applied in the development of new catalysts and materials, as well as in the synthesis of deuterated compounds for various industrial applications.
Mechanism of Action
The mechanism of action of p-toluidine-D3 (methyl-D3) involves its interaction with molecular targets and pathways similar to those of p-toluidine. The deuterium labeling allows for the tracking of the compound in various reactions and processes. The amino group in p-toluidine-D3 (methyl-D3) can participate in hydrogen bonding and electrophilic substitution reactions, influencing its reactivity and interactions with other molecules.
Comparison with Similar Compounds
P-Toluidine-D3 (methyl-D3) can be compared with other deuterated and non-deuterated aromatic amines, such as:
P-Toluidine: The non-deuterated analog with similar chemical properties but without the isotopic labeling.
O-Toluidine and M-Toluidine: Isomers of p-toluidine with the amino group at different positions on the benzene ring.
Aniline: A simpler aromatic amine without the methyl group, used as a reference compound in various studies.
The uniqueness of p-toluidine-D3 (methyl-D3) lies in its deuterium labeling, which provides valuable insights into reaction mechanisms and pathways that are not easily accessible with non-deuterated compounds.
Properties
IUPAC Name |
4-(trideuteriomethyl)aniline | |
---|---|---|
Source | PubChem | |
URL | https://pubchem.ncbi.nlm.nih.gov | |
Description | Data deposited in or computed by PubChem | |
InChI |
InChI=1S/C7H9N/c1-6-2-4-7(8)5-3-6/h2-5H,8H2,1H3/i1D3 | |
Source | PubChem | |
URL | https://pubchem.ncbi.nlm.nih.gov | |
Description | Data deposited in or computed by PubChem | |
InChI Key |
RZXMPPFPUUCRFN-FIBGUPNXSA-N | |
Source | PubChem | |
URL | https://pubchem.ncbi.nlm.nih.gov | |
Description | Data deposited in or computed by PubChem | |
Canonical SMILES |
CC1=CC=C(C=C1)N | |
Source | PubChem | |
URL | https://pubchem.ncbi.nlm.nih.gov | |
Description | Data deposited in or computed by PubChem | |
Isomeric SMILES |
[2H]C([2H])([2H])C1=CC=C(C=C1)N | |
Source | PubChem | |
URL | https://pubchem.ncbi.nlm.nih.gov | |
Description | Data deposited in or computed by PubChem | |
Molecular Formula |
C7H9N | |
Source | PubChem | |
URL | https://pubchem.ncbi.nlm.nih.gov | |
Description | Data deposited in or computed by PubChem | |
Molecular Weight |
110.17 g/mol | |
Source | PubChem | |
URL | https://pubchem.ncbi.nlm.nih.gov | |
Description | Data deposited in or computed by PubChem | |
Synthesis routes and methods
Procedure details
Retrosynthesis Analysis
AI-Powered Synthesis Planning: Our tool employs the Template_relevance Pistachio, Template_relevance Bkms_metabolic, Template_relevance Pistachio_ringbreaker, Template_relevance Reaxys, Template_relevance Reaxys_biocatalysis model, leveraging a vast database of chemical reactions to predict feasible synthetic routes.
One-Step Synthesis Focus: Specifically designed for one-step synthesis, it provides concise and direct routes for your target compounds, streamlining the synthesis process.
Accurate Predictions: Utilizing the extensive PISTACHIO, BKMS_METABOLIC, PISTACHIO_RINGBREAKER, REAXYS, REAXYS_BIOCATALYSIS database, our tool offers high-accuracy predictions, reflecting the latest in chemical research and data.
Strategy Settings
Precursor scoring | Relevance Heuristic |
---|---|
Min. plausibility | 0.01 |
Model | Template_relevance |
Template Set | Pistachio/Bkms_metabolic/Pistachio_ringbreaker/Reaxys/Reaxys_biocatalysis |
Top-N result to add to graph | 6 |
Feasible Synthetic Routes
Disclaimer and Information on In-Vitro Research Products
Please be aware that all articles and product information presented on BenchChem are intended solely for informational purposes. The products available for purchase on BenchChem are specifically designed for in-vitro studies, which are conducted outside of living organisms. In-vitro studies, derived from the Latin term "in glass," involve experiments performed in controlled laboratory settings using cells or tissues. It is important to note that these products are not categorized as medicines or drugs, and they have not received approval from the FDA for the prevention, treatment, or cure of any medical condition, ailment, or disease. We must emphasize that any form of bodily introduction of these products into humans or animals is strictly prohibited by law. It is essential to adhere to these guidelines to ensure compliance with legal and ethical standards in research and experimentation.