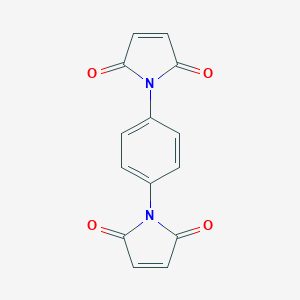
N,N'-1,4-Phenylenedimaleimide
Overview
Description
Phenyl-1,4-bismaleimide is a compound belonging to the bismaleimide family, known for its high thermal stability, mechanical strength, and excellent chemical resistance. These properties make it a valuable material in various high-performance applications, including aerospace, electronics, and advanced composites.
Mechanism of Action
Target of Action
N,N’-1,4-Phenylenedimaleimide, also known as N,N’-4-Phenylenedimaleimide, is a bifunctional cross-linking reagent . Its primary targets are proteins, specifically those containing sulfhydryl groups .
Mode of Action
The compound interacts with its targets through a process known as cross-linking. It forms covalent bonds with the sulfhydryl groups of proteins, leading to the formation of dimers . This interaction results in changes to the protein structure and function .
Biochemical Pathways
It’s known that the compound plays a role in the polymerization of actin, a protein that forms microfilaments . The cross-linking of actin by N,N’-1,4-Phenylenedimaleimide leads to the formation of lower dimers (LD), which are involved in the nucleation step of actin polymerization .
Pharmacokinetics
Given its molecular weight of 26822 , it’s likely that the compound has good bioavailability.
Result of Action
The cross-linking of proteins by N,N’-1,4-Phenylenedimaleimide can result in changes to the proteins’ structure and function . In the case of actin, the formation of lower dimers leads to the polymerization of the protein, affecting cell movement and structure .
Action Environment
The action of N,N’-1,4-Phenylenedimaleimide can be influenced by various environmental factors. For instance, the compound’s reactivity with sulfhydryl groups is pH-dependent, with optimal activity observed at alkaline pH levels . Additionally, the stability of the compound may be affected by temperature and light exposure.
Biochemical Analysis
Biochemical Properties
N,N’-1,4-Phenylenedimaleimide plays a crucial role in biochemical reactions due to its bifunctional nature, allowing it to form covalent bonds with thiol groups in proteins. This compound interacts with various enzymes, proteins, and other biomolecules, facilitating the cross-linking of proteins. For instance, it has been used to cross-link F-actin through lysine-191 and cysteine-374 . The nature of these interactions is primarily covalent, forming stable bonds that can withstand various biochemical assays.
Temporal Effects in Laboratory Settings
In laboratory settings, the effects of N,N’-1,4-Phenylenedimaleimide can change over time. The stability and degradation of this compound are critical factors that influence its long-term effects on cellular function. Studies have shown that N,N’-1,4-Phenylenedimaleimide is relatively stable under standard laboratory conditions, but its reactivity can decrease over time due to hydrolysis and other degradation processes . Long-term exposure to this compound can lead to sustained changes in protein structure and function, impacting cellular processes.
Preparation Methods
Synthetic Routes and Reaction Conditions: Phenyl-1,4-bismaleimide can be synthesized through the imidization of bisamic acid derivatives. The process typically involves the reaction of maleic anhydride with diamines to form bisamic acids, which are then cyclized to form bismaleimides under thermal conditions .
Industrial Production Methods: In industrial settings, the production of phenyl-1,4-bismaleimide often involves the use of high-purity raw materials and controlled reaction environments to ensure consistent quality. The process may include steps such as solvent extraction, purification, and thermal curing to achieve the desired product properties .
Chemical Reactions Analysis
Types of Reactions: Phenyl-1,4-bismaleimide undergoes various chemical reactions, including:
Polymerization: It can polymerize through a free-radical mechanism, forming highly crosslinked thermosetting polymers.
Michael Addition: This reaction involves the addition of nucleophiles to the maleimide double bonds, leading to chain extension and reduced brittleness.
Common Reagents and Conditions:
Polymerization: Typically initiated by heat or free-radical initiators.
Michael Addition: Common reagents include primary or secondary amines, often conducted at elevated temperatures.
Major Products:
Polymerization: Results in highly crosslinked thermosetting polymers.
Michael Addition: Produces chain-extended polymers with improved toughness.
Scientific Research Applications
Phenyl-1,4-bismaleimide has a wide range of applications in scientific research:
Comparison with Similar Compounds
4,4’-Bismaleimidodiphenylmethane: Known for its high thermal stability and mechanical properties.
2,2’-Diallylbisphenol A modified bismaleimide: Offers improved processability and lower brittleness.
Uniqueness: Phenyl-1,4-bismaleimide stands out due to its specific molecular structure, which provides a unique balance of thermal stability, mechanical strength, and chemical resistance. Its ability to form highly crosslinked networks without the need for additional catalysts or initiators further distinguishes it from other bismaleimides .
Biological Activity
N,N'-1,4-Phenylenedimaleimide (pPDM) is a bifunctional maleimide compound with the molecular formula . It is primarily recognized for its role as a cross-linking agent in biochemical research, particularly in studies involving protein interactions and structural biology. This article delves into the biological activity of pPDM, highlighting its mechanisms of action, applications in scientific research, and relevant case studies.
pPDM functions as a bifunctional cross-linker, primarily targeting thiol groups in proteins. The maleimide moiety reacts selectively with free sulfhydryl groups, leading to the formation of stable thioether bonds. This property is exploited in various biochemical applications, including:
- Protein Cross-Linking : Facilitates the study of protein conformations and interactions.
- Structural Analysis : Used to stabilize protein complexes for crystallography and other structural determination techniques.
Reaction Mechanism
The reaction of pPDM with thiol groups can be summarized as follows:
where R represents the protein or peptide backbone.
1. Protein Interaction Studies
Research has demonstrated that pPDM can effectively cross-link myosin heavy chains, providing insights into the dynamics and structural flexibility of muscle proteins. For instance, a study indicated that treatment with pPDM resulted in significant cross-linking of myosin subfragment 1 (S1), revealing critical information about the conformational states of myosin during muscle contraction .
2. Effects on Actin Polymerization
pPDM has been shown to influence actin polymerization dynamics. In experiments where actin was treated with pPDM, rapid formation of lower dimers was observed, suggesting that pPDM can enhance nucleation steps in actin filament assembly . This finding is pivotal for understanding cytoskeletal dynamics and cellular motility.
3. Cross-Linking G Proteins
In neuronal studies, pPDM was utilized to cross-link heterotrimeric G proteins within rat brain synaptoneurosomes. This application highlighted the utility of pPDM in elucidating protein-protein interactions critical for neurotransmission processes .
Case Study 1: Myosin Cross-Linking
A detailed investigation into myosin's ATPase activity revealed that modification with pPDM significantly altered the enzyme's kinetics. The study characterized how pPDM binding affected the interaction between myosin and actin filaments under various nucleotide conditions, providing a deeper understanding of muscle contraction mechanisms .
Case Study 2: Actin Dynamics
Another study focused on the role of sulfhydryl groups in actin polymerization, demonstrating that pPDM could enhance filament stability. The results indicated that the presence of pPDM led to increased resistance against depolymerization under stress conditions, which is essential for maintaining cytoskeletal integrity in cells .
Summary of Findings
The biological activity of this compound reveals its significant role as a cross-linking agent in biochemical research. It facilitates:
- Protein Structural Studies : Enhances understanding of protein conformations.
- Actin Dynamics : Influences polymerization and stability.
- Neurotransmission Research : Elucidates protein interactions critical for neuronal function.
Data Table: Summary of Biological Activities
Properties
IUPAC Name |
1-[4-(2,5-dioxopyrrol-1-yl)phenyl]pyrrole-2,5-dione | |
---|---|---|
Source | PubChem | |
URL | https://pubchem.ncbi.nlm.nih.gov | |
Description | Data deposited in or computed by PubChem | |
InChI |
InChI=1S/C14H8N2O4/c17-11-5-6-12(18)15(11)9-1-2-10(4-3-9)16-13(19)7-8-14(16)20/h1-8H | |
Source | PubChem | |
URL | https://pubchem.ncbi.nlm.nih.gov | |
Description | Data deposited in or computed by PubChem | |
InChI Key |
AQGZJQNZNONGKY-UHFFFAOYSA-N | |
Source | PubChem | |
URL | https://pubchem.ncbi.nlm.nih.gov | |
Description | Data deposited in or computed by PubChem | |
Canonical SMILES |
C1=CC(=CC=C1N2C(=O)C=CC2=O)N3C(=O)C=CC3=O | |
Source | PubChem | |
URL | https://pubchem.ncbi.nlm.nih.gov | |
Description | Data deposited in or computed by PubChem | |
Molecular Formula |
C14H8N2O4 | |
Source | PubChem | |
URL | https://pubchem.ncbi.nlm.nih.gov | |
Description | Data deposited in or computed by PubChem | |
DSSTOX Substance ID |
DTXSID70186446 | |
Record name | N,N'-4-Phenylenedimaleimide | |
Source | EPA DSSTox | |
URL | https://comptox.epa.gov/dashboard/DTXSID70186446 | |
Description | DSSTox provides a high quality public chemistry resource for supporting improved predictive toxicology. | |
Molecular Weight |
268.22 g/mol | |
Source | PubChem | |
URL | https://pubchem.ncbi.nlm.nih.gov | |
Description | Data deposited in or computed by PubChem | |
CAS No. |
3278-31-7 | |
Record name | 1,4-Phenylenebismaleimide | |
Source | CAS Common Chemistry | |
URL | https://commonchemistry.cas.org/detail?cas_rn=3278-31-7 | |
Description | CAS Common Chemistry is an open community resource for accessing chemical information. Nearly 500,000 chemical substances from CAS REGISTRY cover areas of community interest, including common and frequently regulated chemicals, and those relevant to high school and undergraduate chemistry classes. This chemical information, curated by our expert scientists, is provided in alignment with our mission as a division of the American Chemical Society. | |
Explanation | The data from CAS Common Chemistry is provided under a CC-BY-NC 4.0 license, unless otherwise stated. | |
Record name | N,N'-4-Phenylenedimaleimide | |
Source | ChemIDplus | |
URL | https://pubchem.ncbi.nlm.nih.gov/substance/?source=chemidplus&sourceid=0003278317 | |
Description | ChemIDplus is a free, web search system that provides access to the structure and nomenclature authority files used for the identification of chemical substances cited in National Library of Medicine (NLM) databases, including the TOXNET system. | |
Record name | 3278-31-7 | |
Source | DTP/NCI | |
URL | https://dtp.cancer.gov/dtpstandard/servlet/dwindex?searchtype=NSC&outputformat=html&searchlist=81257 | |
Description | The NCI Development Therapeutics Program (DTP) provides services and resources to the academic and private-sector research communities worldwide to facilitate the discovery and development of new cancer therapeutic agents. | |
Explanation | Unless otherwise indicated, all text within NCI products is free of copyright and may be reused without our permission. Credit the National Cancer Institute as the source. | |
Record name | N,N'-4-Phenylenedimaleimide | |
Source | EPA DSSTox | |
URL | https://comptox.epa.gov/dashboard/DTXSID70186446 | |
Description | DSSTox provides a high quality public chemistry resource for supporting improved predictive toxicology. | |
Record name | N,N'-p-phenylenedimaleimide | |
Source | European Chemicals Agency (ECHA) | |
URL | https://echa.europa.eu/substance-information/-/substanceinfo/100.019.919 | |
Description | The European Chemicals Agency (ECHA) is an agency of the European Union which is the driving force among regulatory authorities in implementing the EU's groundbreaking chemicals legislation for the benefit of human health and the environment as well as for innovation and competitiveness. | |
Explanation | Use of the information, documents and data from the ECHA website is subject to the terms and conditions of this Legal Notice, and subject to other binding limitations provided for under applicable law, the information, documents and data made available on the ECHA website may be reproduced, distributed and/or used, totally or in part, for non-commercial purposes provided that ECHA is acknowledged as the source: "Source: European Chemicals Agency, http://echa.europa.eu/". Such acknowledgement must be included in each copy of the material. ECHA permits and encourages organisations and individuals to create links to the ECHA website under the following cumulative conditions: Links can only be made to webpages that provide a link to the Legal Notice page. | |
Record name | N,N'-4-PHENYLENEDIMALEIMIDE | |
Source | FDA Global Substance Registration System (GSRS) | |
URL | https://gsrs.ncats.nih.gov/ginas/app/beta/substances/BEC7P1E6J1 | |
Description | The FDA Global Substance Registration System (GSRS) enables the efficient and accurate exchange of information on what substances are in regulated products. Instead of relying on names, which vary across regulatory domains, countries, and regions, the GSRS knowledge base makes it possible for substances to be defined by standardized, scientific descriptions. | |
Explanation | Unless otherwise noted, the contents of the FDA website (www.fda.gov), both text and graphics, are not copyrighted. They are in the public domain and may be republished, reprinted and otherwise used freely by anyone without the need to obtain permission from FDA. Credit to the U.S. Food and Drug Administration as the source is appreciated but not required. | |
Retrosynthesis Analysis
AI-Powered Synthesis Planning: Our tool employs the Template_relevance Pistachio, Template_relevance Bkms_metabolic, Template_relevance Pistachio_ringbreaker, Template_relevance Reaxys, Template_relevance Reaxys_biocatalysis model, leveraging a vast database of chemical reactions to predict feasible synthetic routes.
One-Step Synthesis Focus: Specifically designed for one-step synthesis, it provides concise and direct routes for your target compounds, streamlining the synthesis process.
Accurate Predictions: Utilizing the extensive PISTACHIO, BKMS_METABOLIC, PISTACHIO_RINGBREAKER, REAXYS, REAXYS_BIOCATALYSIS database, our tool offers high-accuracy predictions, reflecting the latest in chemical research and data.
Strategy Settings
Precursor scoring | Relevance Heuristic |
---|---|
Min. plausibility | 0.01 |
Model | Template_relevance |
Template Set | Pistachio/Bkms_metabolic/Pistachio_ringbreaker/Reaxys/Reaxys_biocatalysis |
Top-N result to add to graph | 6 |
Feasible Synthetic Routes
Q1: How does N,N'-1,4-Phenylenedimaleimide (p-PDM) interact with proteins and what are the downstream effects?
A: p-PDM acts as a cross-linking agent by forming covalent bonds with cysteine residues present in proteins. This cross-linking can have various downstream effects depending on the target protein. For instance, p-PDM inhibits the function of transducin by cross-linking cysteine residues in its alpha subunit, thereby disrupting its interaction with the beta-gamma subunit and hindering downstream signaling. [] Similarly, p-PDM treatment of G-proteins in synaptoneurosomes results in the formation of large cross-linked structures, suggesting an impact on their oligomerization state and potential influence on signal transduction pathways. []
Q2: Can you provide details on the structural characterization of this compound?
A2: this compound consists of a phenyl ring core with two maleimide groups attached at opposite ends (para position).
Q3: How does this compound affect actin polymerization and filament structure?
A: p-PDM's interaction with actin reveals insights into actin filament structure and the mechanism of proteins like gelsolin. Research shows that p-PDM cross-links actin monomers into a specific "lower dimer," indicating an antiparallel orientation of the monomers within this dimer. [] This finding challenges the previously held assumption that actin monomers in the gelsolin complex are arranged like F-actin. Additionally, p-PDM inhibits the formation of the "upper dimer" usually observed in cross-linked F-actin, further highlighting gelsolin's role in influencing actin filament structure. [] In contrast to gelsolin, the gonococcal porin P.IB, when interacting with actin in the presence of p-PDM, allows for cross-linking, leading to the formation of fragmented F-actin-like filaments. [] This suggests that P.IB modifies the arrangement of actin monomers, making them accessible to p-PDM cross-linking.
Q4: What is the significance of this compound in studying G protein oligomerization?
A: p-PDM has been instrumental in investigating the oligomerization state of G proteins. Studies using p-PDM cross-linking provided evidence for the existence of G protein oligomers, particularly for G(o)alpha. [] The observation that GTPgammaS activation inhibits p-PDM cross-linking of G(o)alpha suggests that this activation leads to the disaggregation of G protein oligomers, supporting the "disaggregation-coupling" theory. [] This finding has implications for understanding the dynamic regulation of G protein signaling pathways.
Q5: Are there any documented applications of this compound in material science?
A: Indeed, p-PDM finds use in synthesizing porous polymers. Research demonstrates its utility in a catalyst-free Diels-Alder reaction with a multicyclopentadiene-functionalized monomer to create a crosslinked polymer (CDAP). [] This polymer's structure can be further modified by exploiting the thermal reversibility of the Diels-Alder linkage, significantly increasing its surface area. [] This example highlights p-PDM's versatility in materials science applications.
Disclaimer and Information on In-Vitro Research Products
Please be aware that all articles and product information presented on BenchChem are intended solely for informational purposes. The products available for purchase on BenchChem are specifically designed for in-vitro studies, which are conducted outside of living organisms. In-vitro studies, derived from the Latin term "in glass," involve experiments performed in controlled laboratory settings using cells or tissues. It is important to note that these products are not categorized as medicines or drugs, and they have not received approval from the FDA for the prevention, treatment, or cure of any medical condition, ailment, or disease. We must emphasize that any form of bodily introduction of these products into humans or animals is strictly prohibited by law. It is essential to adhere to these guidelines to ensure compliance with legal and ethical standards in research and experimentation.