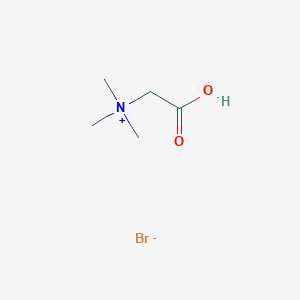
(Carboxymethyl)trimethylammonium bromide
- Click on QUICK INQUIRY to receive a quote from our team of experts.
- With the quality product at a COMPETITIVE price, you can focus more on your research.
Overview
Description
(Carboxymethyl)trimethylammonium bromide is a quaternary ammonium compound with the molecular formula C5H12BrNO2. It is known for its surfactant properties and is widely used in various industrial and scientific applications. The compound is characterized by the presence of a carboxymethyl group attached to a trimethylammonium moiety, with bromide as the counterion.
Preparation Methods
Synthetic Routes and Reaction Conditions: The synthesis of (Carboxymethyl)trimethylammonium bromide typically involves the reaction of trimethylamine with bromoacetic acid. The reaction proceeds as follows:
Reactants: Trimethylamine and bromoacetic acid.
Reaction Conditions: The reaction is carried out in an aqueous medium at a controlled temperature, usually around 50-60°C.
Procedure: Trimethylamine is added to a solution of bromoacetic acid, and the mixture is stirred until the reaction is complete. The product is then isolated by precipitation or crystallization.
Industrial Production Methods: In industrial settings, the production of this compound is scaled up using continuous flow reactors. This allows for better control over reaction conditions and higher yields. The process involves:
Continuous Flow Reactors: These reactors ensure consistent mixing and temperature control.
Purification: The crude product is purified using techniques such as recrystallization or chromatography to obtain high-purity this compound.
Chemical Reactions Analysis
Types of Reactions: (Carboxymethyl)trimethylammonium bromide undergoes various chemical reactions, including:
Substitution Reactions: The bromide ion can be substituted by other nucleophiles, such as hydroxide or chloride ions.
Oxidation and Reduction: The compound can participate in redox reactions, although these are less common.
Common Reagents and Conditions:
Substitution Reactions: Typically carried out in aqueous or alcoholic solutions with reagents like sodium hydroxide or hydrochloric acid.
Oxidation and Reduction: These reactions may require specific catalysts or conditions, such as the presence of oxidizing agents like potassium permanganate.
Major Products:
Substitution Products: Depending on the nucleophile, products such as (Carboxymethyl)trimethylammonium chloride can be formed.
Oxidation Products: These may include various oxidized derivatives of the compound.
Scientific Research Applications
(Carboxymethyl)trimethylammonium bromide has a wide range of applications in scientific research:
Chemistry: Used as a surfactant and phase transfer catalyst in organic synthesis.
Biology: Employed in the extraction and purification of nucleic acids, particularly in the CTAB method for DNA extraction.
Medicine: Investigated for its potential antimicrobial properties and use in drug delivery systems.
Industry: Utilized in the formulation of personal care products, detergents, and as an antistatic agent in textiles.
Mechanism of Action
The mechanism of action of (Carboxymethyl)trimethylammonium bromide is primarily based on its surfactant properties. The compound can disrupt cell membranes by integrating into the lipid bilayer, leading to increased permeability and cell lysis. This makes it effective as an antimicrobial agent. Additionally, its ability to form micelles and solubilize hydrophobic compounds is leveraged in various applications, such as drug delivery and nucleic acid extraction.
Comparison with Similar Compounds
Cetyltrimethylammonium Bromide (CTAB): Another quaternary ammonium compound with similar surfactant properties, widely used in DNA extraction and as an antiseptic.
Tetrabutylammonium Bromide: Used as a phase transfer catalyst in organic synthesis.
Benzalkonium Chloride: A quaternary ammonium compound with strong antimicrobial properties, commonly used in disinfectants and antiseptics.
Uniqueness: (Carboxymethyl)trimethylammonium bromide is unique due to the presence of the carboxymethyl group, which imparts additional solubility and reactivity compared to other quaternary ammonium compounds. This makes it particularly useful in applications requiring high solubility and specific reactivity, such as in biological and medical research.
Properties
CAS No. |
5938-06-7 |
---|---|
Molecular Formula |
C5H12BrNO2 |
Molecular Weight |
198.06 g/mol |
IUPAC Name |
carboxymethyl(trimethyl)azanium;bromide |
InChI |
InChI=1S/C5H11NO2.BrH/c1-6(2,3)4-5(7)8;/h4H2,1-3H3;1H |
InChI Key |
ZCCXZMHMPCCJIG-UHFFFAOYSA-N |
Canonical SMILES |
C[N+](C)(C)CC(=O)O.[Br-] |
Origin of Product |
United States |
Disclaimer and Information on In-Vitro Research Products
Please be aware that all articles and product information presented on BenchChem are intended solely for informational purposes. The products available for purchase on BenchChem are specifically designed for in-vitro studies, which are conducted outside of living organisms. In-vitro studies, derived from the Latin term "in glass," involve experiments performed in controlled laboratory settings using cells or tissues. It is important to note that these products are not categorized as medicines or drugs, and they have not received approval from the FDA for the prevention, treatment, or cure of any medical condition, ailment, or disease. We must emphasize that any form of bodily introduction of these products into humans or animals is strictly prohibited by law. It is essential to adhere to these guidelines to ensure compliance with legal and ethical standards in research and experimentation.