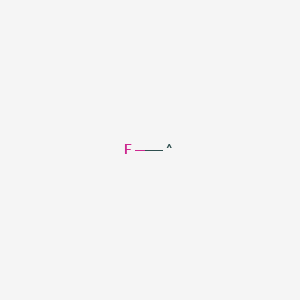
Fluoromethyl radical
- Click on QUICK INQUIRY to receive a quote from our team of experts.
- With the quality product at a COMPETITIVE price, you can focus more on your research.
Overview
Description
The fluoromethyl radical (CH₂F) is a highly reactive, open-shell species characterized by a single unpaired electron on the carbon atom adjacent to the fluorine substituent. Its reactivity stems from the electron-withdrawing nature of fluorine, which polarizes the C–F bond and lowers the activation energy for radical-mediated transformations. CH₂F has gained prominence in synthetic chemistry due to its ability to introduce the pharmaceutically valuable fluoromethyl (CH₂F) group into organic molecules. This group serves as a bioisostere for methyl (CH₃) or hydroxymethyl (CH₂OH) groups, enhancing metabolic stability and lipophilicity in drug candidates .
Recent advancements in CH₂F radical generation utilize halogen atom transfer (XAT) activation of commercially available fluoroiodomethane (CH₂FI) under visible light, eliminating the need for strong reductants or expensive photocatalysts. For example, tris(trimethylsilyl)silane [(Me₃Si)₃Si–H] acts synergistically as a hydrogen atom transfer (HAT) reagent and XAT agent, enabling chain-propagating radical fluoromethylation of iminium ions and electron-deficient alkenes . This method provides access to α-fluoromethyl amines and β-fluoromethyl esters, which are challenging to synthesize via traditional ionic pathways .
Preparation Methods
The generation of fluoromethyl radicals typically involves the activation of fluoroiodomethane (CH₂FI) via halogen atom transfer. This process can be mediated by visible light and tris(trimethylsilyl)silane, which acts as both a hydrogen and halogen atom transfer reagent. This method is advantageous as it avoids the need for metal catalysts and allows for the formation of C(sp³)–CH₂F bonds through a radical chain process . The reaction conditions are mild, and the process can be carried out at room temperature, making it suitable for industrial applications.
Chemical Reactions Analysis
Fluoromethyl radicals participate in a variety of chemical reactions, including:
Addition Reactions: Fluoromethyl radicals can add to unsaturated carbon-carbon or carbon-heteroatom bonds, forming new C–CH₂F bonds.
Substitution Reactions: These radicals can also undergo substitution reactions, where the fluoromethyl group replaces a hydrogen atom or another substituent on an organic molecule.
Oxidation and Reduction: While less common, fluoromethyl radicals can participate in oxidation and reduction reactions under specific conditions.
Common reagents used in these reactions include fluoroiodomethane, tris(trimethylsilyl)silane, and various unsaturated substrates such as alkenes and alkynes. The major products formed from these reactions are fluoromethylated compounds, which serve as valuable intermediates in synthetic and medicinal chemistry .
Scientific Research Applications
The fluoromethyl (CH2F) group is a motif of pharmaceutical importance, and fluoromethyl radicals have applications inVisible light-mediated radical fluoromethylation .
Scientific Research Applications
- Visible Light-Mediated Radical Fluoromethylation Visible light and tris(trimethylsilyl)silane orchestrate a fluoromethylation strategy based on the generation of fluoromethyl radicals from commercially available fluoroiodomethane via halogen atom transfer . Tris(trimethylsilyl)silane serves as both a hydrogen- and halogen atom transfer reagent to facilitate the formation of C(sp3)–CH2F bonds via a radical chain process . This metal- and photocatalyst-free transformation is applicable in the multicomponent synthesis of complex α-fluoromethyl amines and amino acid derivatives via radical addition to in situ-formed iminium ions, and the construction of β-fluoromethyl esters and amides from electron-deficient alkene acceptors .
- Hydrofluoromethylation of Alkenes Fluoroiodomethane has applications as an electrophilic or nucleophilic fluoromethylation reagent, including in cross-coupling reactions . [18F]CH2FI is suited for [18F]CH2F radical chemistry . Ibrutinib, an estrone, a tyrosine, and an ethacrynic acid derivative underwent 18F-hydrofluoromethylation in radiochemical yields up to 81% .
- Organic Photocatalytic Generation Visible light-mediated three-component monofluoromethylation/acylation of styrene derivatives employing NHC and organic photocatalysis .
Case Studies
Mechanism of Action
The mechanism by which fluoromethyl radicals exert their effects involves the formation of new C–CH₂F bonds through radical addition or substitution reactions. The process is initiated by the abstraction of a halogen atom from fluoroiodomethane by a stable silyl radical, generating the fluoromethyl radical. This radical then reacts with unsaturated substrates to form the desired fluoromethylated products . The high reactivity of the this compound allows for the efficient formation of these products under mild conditions.
Comparison with Similar Compounds
Comparison with Similar Fluorinated Radicals
Difluoromethyl Radical (CF₂H)
The dithis compound (CF₂H) shares structural similarities with CH₂F but exhibits distinct electronic properties due to the presence of two fluorine atoms. Key differences include:
- Generation: CF₂H is typically generated via single-electron transfer (SET) reduction of sulfonyl-based precursors (e.g., PhSO₂CF₂H), which have highly negative reduction potentials (E₍red₎ ≈ −2.43 V vs. SCE). This necessitates strong reductants like Mn powder or UV irradiation, limiting functional group compatibility .
- Reactivity : CF₂H is more electrophilic than CH₂F, favoring addition to electron-rich alkenes. However, its lower nucleophilicity restricts applications in polar mismatch scenarios.
- Applications : CF₂H is employed in the synthesis of difluoromethyl thioethers and agrochemicals, though its use in multicomponent reactions remains underdeveloped compared to CH₂F .
Example : Photoredox-catalyzed CF₂H radical addition to thiols yields difluoromethyl sulfides, leveraging the σ-hole effect of phosphonium salts (e.g., CF₂HPPh₃⁺I⁻) .
Trithis compound (CF₃)
The trithis compound (CF₃) is the most electronegative among fluorinated methyl radicals, with widespread applications in pharmaceuticals and materials science. Key distinctions from CH₂F include:
- Generation: CF₃ is commonly generated from electrophilic reagents (e.g., Togni’s reagent or Umemoto’s reagent) via photoredox catalysis. These precursors have moderate reduction potentials (E₍red₎ ≈ −1.1 to −1.5 V vs. SCE), enabling activation with Ru(bpy)₃²⁺ or Ir(ppy)₃ photocatalysts under visible light .
- Reactivity : CF₃ exhibits strong electron-withdrawing effects, promoting trifluoromethylation of electron-rich arenes and alkenes. Its high stability reduces undesired side reactions, such as radical recombination.
- Applications: CF₃ is widely used in trifluoromethylative difunctionalization of alkenes (e.g., oxy- and amino-trifluoromethylation) to access CF₃-containing alcohols and amines .
Example : Ru(bpy)₃²⁺-catalyzed trifluoromethylation of styrenes with Togni’s reagent yields β-trifluoromethyl ketones via a radical-polar crossover mechanism .
Comparative Data Table
Q & A
Basic Research Questions
Q. How are fluoromethyl radicals generated in experimental settings, and what factors influence their stability?
Fluoromethyl radicals (CH₂F•) are typically generated via halogen atom transfer (XAT) from fluoroiodomethane (CH₂FI) using visible light and tris(trimethylsilyl)silane [(Me₃Si)₃Si–H]. This method avoids the need for strongly reducing reagents by leveraging a radical chain mechanism: (Me₃Si)₃Si–H facilitates both hydrogen atom transfer (HAT) and XAT, enabling continuous radical generation . Stability is influenced by reaction conditions (e.g., solvent, temperature) and the presence of electron-deficient acceptors, which stabilize the radical through polarity-matched interactions .
Q. What spectroscopic techniques are used to detect and characterize fluoromethyl radicals?
Microwave spectroscopy is a primary method for detecting CH₂F•, as demonstrated by the observation of hyperfine splitting in its ground and excited vibrational states. The radical’s planar geometry (B₁ symmetry) and out-of-plane bending mode (ν₄ at ~300 cm⁻¹) were confirmed via precise measurement of a-type R-branch transitions . Electron paramagnetic resonance (EPR) and time-resolved infrared spectroscopy are also used to monitor radical intermediates in reaction pathways .
Q. How do fluoromethyl radicals interact with common atmospheric species like O₂ and OH?
At high temperatures (e.g., in flames), CH₂F• reacts with O₂ via direct O–O bond cleavage to form fluoromethoxy radicals (CH₂FO•) and oxygen atoms. Rate constants for these reactions are extrapolated from analogous •CF₃ data but require adjustment for enthalpy differences. Reactions with OH radicals follow similar kinetics to •CH₃, though uncertainties persist due to limited experimental data at elevated temperatures .
Advanced Research Questions
Q. What strategies address contradictions in kinetic data for fluoromethyl radical reactions, particularly under pyrolytic vs. ambient conditions?
Discrepancies arise from extrapolating low-temperature (300–600 K) rate measurements to high-temperature flame conditions. For example, •CH₂F + O₂ rate constants estimated via RRKM theory assume no reverse barrier, but steady-state approximations for CH₂FO• formation may not hold under pyrolysis. Refinement requires benchmarking against shock-tube experiments or laser-induced fluorescence (LIF) data to validate non-Arrhenius behavior .
Q. How can multicomponent reactions be designed to incorporate fluoromethyl radicals into complex amines or esters?
Visible light-mediated XAT enables three-component carbonyl-fluoromethylative amination. Iminium ions, generated in situ from aldehydes and amines, react with CH₂F• to form α-fluoromethyl alkylamines. Similarly, electron-deficient alkenes undergo hydrofluoromethylation to yield β-fluoromethyl esters/amides. Key to success is the use of (Me₃Si)₃Si–H to sustain the radical chain and suppress side reactions .
Q. What thermodynamic parameters govern the regioselectivity of this compound addition to unsaturated substrates?
Polarity matching dictates regioselectivity: CH₂F• (electrophilic) preferentially adds to electron-deficient alkenes (e.g., acrylates) at the β-position. Radical stabilization energy (RSE) and bond dissociation energies (BDEs) further influence pathways. For example, the C–I BDE in CH₂FI (~54 kcal/mol) is critical for XAT efficiency, while the C–H BDE in (Me₃Si)₃Si–H (~84 kcal/mol) ensures rapid HAT termination .
Q. How do computational methods resolve ambiguities in this compound reaction mechanisms?
Density functional theory (DFT) calculations model transition states for XAT and HAT steps, revealing that silicon-centered radicals [(Me₃Si)₃Si•] drive chain propagation. RRKM/master equation simulations predict pressure-dependent branching ratios for •CH₂F + •CH₃ reactions (HF elimination vs. stabilization), aiding mechanism refinement .
Q. Methodological Guidance
Q. What protocols ensure reproducibility in this compound trapping experiments?
- Use degassed solvents to minimize radical quenching by O₂.
- Optimize light intensity (450–470 nm) for XAT activation .
- Characterize products via ¹⁹F NMR (δ ~ -140 to -160 ppm for CH₂F groups) and high-resolution mass spectrometry (HRMS) .
Q. How should researchers handle discrepancies between experimental and theoretical rate constants?
Properties
CAS No. |
3744-29-4 |
---|---|
Molecular Formula |
CH2F |
Molecular Weight |
33.025 g/mol |
InChI |
InChI=1S/CH2F/c1-2/h1H2 |
InChI Key |
VUWZPRWSIVNGKG-UHFFFAOYSA-N |
Canonical SMILES |
[CH2]F |
Origin of Product |
United States |
Disclaimer and Information on In-Vitro Research Products
Please be aware that all articles and product information presented on BenchChem are intended solely for informational purposes. The products available for purchase on BenchChem are specifically designed for in-vitro studies, which are conducted outside of living organisms. In-vitro studies, derived from the Latin term "in glass," involve experiments performed in controlled laboratory settings using cells or tissues. It is important to note that these products are not categorized as medicines or drugs, and they have not received approval from the FDA for the prevention, treatment, or cure of any medical condition, ailment, or disease. We must emphasize that any form of bodily introduction of these products into humans or animals is strictly prohibited by law. It is essential to adhere to these guidelines to ensure compliance with legal and ethical standards in research and experimentation.