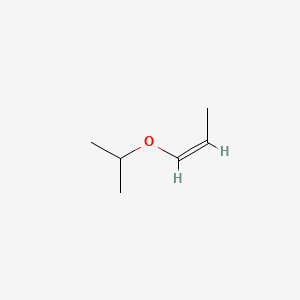
cis-1-Propenyl isopropyl ether
- Click on QUICK INQUIRY to receive a quote from our team of experts.
- With the quality product at a COMPETITIVE price, you can focus more on your research.
Overview
Description
cis-1-Propenyl isopropyl ether: is an organic compound with the molecular formula C6H12O. It is an ether, characterized by an oxygen atom connected to two alkyl or aryl groups. This compound is known for its unique structural configuration, where the propenyl group is in the cis configuration relative to the isopropyl group.
Preparation Methods
Synthetic Routes and Reaction Conditions: The synthesis of cis-1-Propenyl isopropyl ether can be achieved through the Williamson ether synthesis. This method involves the reaction of an alkoxide ion with a primary alkyl halide or tosylate in an S_N2 reaction. The alkoxide ion is typically prepared by reacting an alcohol with a strong base such as sodium hydride .
Industrial Production Methods: Industrial production of ethers like this compound often involves similar synthetic routes but on a larger scale. The process may include the use of continuous flow reactors to ensure efficient mixing and reaction control.
Chemical Reactions Analysis
Types of Reactions:
Acidic Cleavage: Ethers, including cis-1-Propenyl isopropyl ether, can undergo cleavage of the C–O bond using strong acids like HBr or HI.
Oxidation and Reduction: Ethers are generally resistant to oxidation and reduction under mild conditions, making them stable solvents in various chemical reactions.
Common Reagents and Conditions:
Acidic Cleavage: Reagents such as HBr or HI are used under acidic conditions to cleave the ether bond.
Oxidation and Reduction: Ethers are stable under most oxidative and reductive conditions, but strong oxidizing agents can potentially break the ether bond.
Major Products:
Acidic Cleavage: The major products are typically an alcohol and an alkyl halide.
Scientific Research Applications
Chemistry: cis-1-Propenyl isopropyl ether is used as a solvent in various organic reactions due to its stability and low reactivity under standard conditions .
Biology and Medicine:
Industry: In the industrial sector, this compound can be used in the formulation of coatings, adhesives, and other materials where a stable, non-reactive solvent is required .
Mechanism of Action
The primary mechanism of action for cis-1-Propenyl isopropyl ether involves its role as a solvent. It can dissolve a wide range of organic compounds, facilitating various chemical reactions. The molecular targets and pathways are primarily related to its physical properties rather than specific biochemical interactions .
Comparison with Similar Compounds
Diethyl Ether: Another common ether with similar solvent properties but different structural configuration.
Methyl tert-Butyl Ether (MTBE): Used as a gasoline additive, it has different industrial applications compared to cis-1-Propenyl isopropyl ether.
Uniqueness: this compound’s unique cis configuration provides distinct physical and chemical properties, such as boiling point and solubility, which can be advantageous in specific applications .
Properties
CAS No. |
4188-64-1 |
---|---|
Molecular Formula |
C6H12O |
Molecular Weight |
100.16 g/mol |
IUPAC Name |
(Z)-1-propan-2-yloxyprop-1-ene |
InChI |
InChI=1S/C6H12O/c1-4-5-7-6(2)3/h4-6H,1-3H3/b5-4- |
InChI Key |
PLGKZYGGZOMZHL-PLNGDYQASA-N |
Isomeric SMILES |
C/C=C\OC(C)C |
Canonical SMILES |
CC=COC(C)C |
Origin of Product |
United States |
Disclaimer and Information on In-Vitro Research Products
Please be aware that all articles and product information presented on BenchChem are intended solely for informational purposes. The products available for purchase on BenchChem are specifically designed for in-vitro studies, which are conducted outside of living organisms. In-vitro studies, derived from the Latin term "in glass," involve experiments performed in controlled laboratory settings using cells or tissues. It is important to note that these products are not categorized as medicines or drugs, and they have not received approval from the FDA for the prevention, treatment, or cure of any medical condition, ailment, or disease. We must emphasize that any form of bodily introduction of these products into humans or animals is strictly prohibited by law. It is essential to adhere to these guidelines to ensure compliance with legal and ethical standards in research and experimentation.