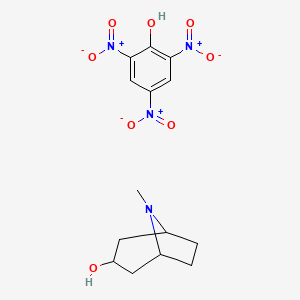
Tropine picrate
- Click on QUICK INQUIRY to receive a quote from our team of experts.
- With the quality product at a COMPETITIVE price, you can focus more on your research.
Overview
Description
Tropine picrate is a chemical compound derived from tropine, a tropane alkaloid, and picric acid. Tropane alkaloids are a class of alkaloids that include compounds such as atropine, scopolamine, and cocaine. These alkaloids are known for their pharmacological properties and are found in various plant families, including Solanaceae and Erythroxylaceae
Preparation Methods
Synthetic Routes and Reaction Conditions
Tropine picrate can be synthesized through the reaction of tropine with picric acid. The reaction typically involves mixing an aqueous solution of tropine with an aqueous solution of picric acid, resulting in the formation of this compound as a precipitate. The reaction is usually carried out at room temperature, and the product is purified by recrystallization .
Industrial Production Methods
This would include the use of industrial reactors, controlled reaction conditions, and purification processes to ensure the quality and yield of the final product .
Chemical Reactions Analysis
Types of Reactions
Tropine picrate undergoes various chemical reactions, including:
Oxidation: Tropine can be oxidized to form tropinone, a key intermediate in the synthesis of other tropane alkaloids.
Reduction: Tropinone can be reduced back to tropine using reducing agents such as sodium borohydride.
Common Reagents and Conditions
Oxidation: Common oxidizing agents include potassium permanganate and chromium trioxide.
Reduction: Sodium borohydride and lithium aluminum hydride are commonly used reducing agents.
Substitution: Various reagents can be used depending on the desired substitution, such as alkyl halides for alkylation reactions.
Major Products Formed
Oxidation: Tropinone
Reduction: Tropine
Substitution: Various tropine derivatives depending on the substituents used.
Scientific Research Applications
Tropine picrate has several applications in scientific research:
Chemistry: Used as a reagent in organic synthesis and as a standard for analytical methods.
Biology: Studied for its interactions with biological molecules and potential effects on biological systems.
Medicine: Investigated for its pharmacological properties and potential therapeutic applications.
Industry: Used in the development of new materials and chemical processes.
Mechanism of Action
The mechanism of action of tropine picrate involves its interaction with various molecular targets. Tropine, as a tropane alkaloid, can interact with muscarinic acetylcholine receptors, leading to anticholinergic effects. This interaction blocks the action of acetylcholine, a neurotransmitter, resulting in various physiological effects . The picrate component may also contribute to the compound’s overall activity, although its specific role is less well understood .
Comparison with Similar Compounds
Similar Compounds
Atropine: Another tropane alkaloid with anticholinergic properties.
Scopolamine: Known for its use in treating motion sickness and nausea.
Cocaine: A stimulant with local anesthetic properties.
Calystegine: A nortropane alkaloid with different biological activities.
Uniqueness
Tropine picrate is unique due to its combination of tropine and picric acid, which may result in distinct chemical and pharmacological properties compared to other tropane alkaloids. Its specific applications and effects are still being explored, making it a compound of interest in various fields of research .
Properties
CAS No. |
6164-68-7 |
---|---|
Molecular Formula |
C14H18N4O8 |
Molecular Weight |
370.31 g/mol |
IUPAC Name |
8-methyl-8-azabicyclo[3.2.1]octan-3-ol;2,4,6-trinitrophenol |
InChI |
InChI=1S/C8H15NO.C6H3N3O7/c1-9-6-2-3-7(9)5-8(10)4-6;10-6-4(8(13)14)1-3(7(11)12)2-5(6)9(15)16/h6-8,10H,2-5H2,1H3;1-2,10H |
InChI Key |
HFBUEWDSKZLEFF-UHFFFAOYSA-N |
Canonical SMILES |
CN1C2CCC1CC(C2)O.C1=C(C=C(C(=C1[N+](=O)[O-])O)[N+](=O)[O-])[N+](=O)[O-] |
Origin of Product |
United States |
Disclaimer and Information on In-Vitro Research Products
Please be aware that all articles and product information presented on BenchChem are intended solely for informational purposes. The products available for purchase on BenchChem are specifically designed for in-vitro studies, which are conducted outside of living organisms. In-vitro studies, derived from the Latin term "in glass," involve experiments performed in controlled laboratory settings using cells or tissues. It is important to note that these products are not categorized as medicines or drugs, and they have not received approval from the FDA for the prevention, treatment, or cure of any medical condition, ailment, or disease. We must emphasize that any form of bodily introduction of these products into humans or animals is strictly prohibited by law. It is essential to adhere to these guidelines to ensure compliance with legal and ethical standards in research and experimentation.