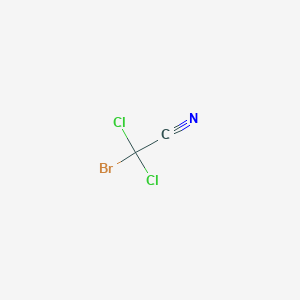
Bromodichloroacetonitrile
Overview
Description
Bromodichloroacetonitrile, also known as this compound, is a useful research compound. Its molecular formula is C2BrCl2N and its molecular weight is 188.84 g/mol. The purity is usually 95%.
BenchChem offers high-quality this compound suitable for many research applications. Different packaging options are available to accommodate customers' requirements. Please inquire for more information about this compound including the price, delivery time, and more detailed information at info@benchchem.com.
Scientific Research Applications
Environmental Impact and Formation
- Haloacetic Acid Formation : A study by Cowman and Singer (1996) explored how bromide ions affect the formation of various haloacetic acids, including bromodichloroacetonitrile, during the chlorination and chloramination of waters containing aquatic humic substances (Cowman & Singer, 1996).
Toxicological Studies
- Comparative Toxicity Analysis : Wei et al. (2020) conducted a comparative toxicology study of haloacetonitriles (HANs), including this compound, evaluating their cytotoxicity, genotoxicity, and thiol reactivity (Wei et al., 2020).
- Toxicology and Carcinogenesis Studies : A technical report by the National Toxicology Program (2015) focused on toxicology studies of bromodichloroacetic acid, a related compound to this compound, in rodents (National Toxicology Program, 2015).
Disinfection By-Products in Water Systems
- By-Products in Cooling Water Systems : Jenner et al. (1997) studied chlorination by-products, including this compound, in cooling water from European coastal power stations (Jenner et al., 1997).
- Water Distribution Systems : Włodyka-Bergier et al. (2014) analyzed the occurrence of various disinfection by-products, such as this compound, in water distribution systems in Krakow, Poland (Włodyka-Bergier et al., 2014).
Applications in Herbicide Resistance
- Bacterial Detoxification Gene for Herbicide Resistance : Stalker et al. (1988) reported on herbicide resistance in transgenic plants expressing a bacterial detoxification gene for bromoxynil, a compound related to this compound (Stalker et al., 1988).
Safety and Hazards
Safety measures for handling Bromodichloroacetonitrile include avoiding dust formation, breathing mist, gas or vapours, and contact with skin and eyes. It is recommended to use personal protective equipment, wear chemical impermeable gloves, ensure adequate ventilation, remove all sources of ignition, evacuate personnel to safe areas, and keep people away from and upwind of spill/leak .
Properties
IUPAC Name |
2-bromo-2,2-dichloroacetonitrile | |
---|---|---|
Source | PubChem | |
URL | https://pubchem.ncbi.nlm.nih.gov | |
Description | Data deposited in or computed by PubChem | |
InChI |
InChI=1S/C2BrCl2N/c3-2(4,5)1-6 | |
Source | PubChem | |
URL | https://pubchem.ncbi.nlm.nih.gov | |
Description | Data deposited in or computed by PubChem | |
InChI Key |
XDJVVCPVQOCPJI-UHFFFAOYSA-N | |
Source | PubChem | |
URL | https://pubchem.ncbi.nlm.nih.gov | |
Description | Data deposited in or computed by PubChem | |
Canonical SMILES |
C(#N)C(Cl)(Cl)Br | |
Source | PubChem | |
URL | https://pubchem.ncbi.nlm.nih.gov | |
Description | Data deposited in or computed by PubChem | |
Molecular Formula |
C2BrCl2N | |
Source | PubChem | |
URL | https://pubchem.ncbi.nlm.nih.gov | |
Description | Data deposited in or computed by PubChem | |
DSSTOX Substance ID |
DTXSID9021508 | |
Record name | Bromodichloroacetonitrile | |
Source | EPA DSSTox | |
URL | https://comptox.epa.gov/dashboard/DTXSID9021508 | |
Description | DSSTox provides a high quality public chemistry resource for supporting improved predictive toxicology. | |
Molecular Weight |
188.84 g/mol | |
Source | PubChem | |
URL | https://pubchem.ncbi.nlm.nih.gov | |
Description | Data deposited in or computed by PubChem | |
CAS No. |
60523-73-1 | |
Record name | Bromodichloroacetonitrile | |
Source | ChemIDplus | |
URL | https://pubchem.ncbi.nlm.nih.gov/substance/?source=chemidplus&sourceid=0060523731 | |
Description | ChemIDplus is a free, web search system that provides access to the structure and nomenclature authority files used for the identification of chemical substances cited in National Library of Medicine (NLM) databases, including the TOXNET system. | |
Record name | Bromodichloroacetonitrile | |
Source | EPA DSSTox | |
URL | https://comptox.epa.gov/dashboard/DTXSID9021508 | |
Description | DSSTox provides a high quality public chemistry resource for supporting improved predictive toxicology. | |
Retrosynthesis Analysis
AI-Powered Synthesis Planning: Our tool employs the Template_relevance Pistachio, Template_relevance Bkms_metabolic, Template_relevance Pistachio_ringbreaker, Template_relevance Reaxys, Template_relevance Reaxys_biocatalysis model, leveraging a vast database of chemical reactions to predict feasible synthetic routes.
One-Step Synthesis Focus: Specifically designed for one-step synthesis, it provides concise and direct routes for your target compounds, streamlining the synthesis process.
Accurate Predictions: Utilizing the extensive PISTACHIO, BKMS_METABOLIC, PISTACHIO_RINGBREAKER, REAXYS, REAXYS_BIOCATALYSIS database, our tool offers high-accuracy predictions, reflecting the latest in chemical research and data.
Strategy Settings
Precursor scoring | Relevance Heuristic |
---|---|
Min. plausibility | 0.01 |
Model | Template_relevance |
Template Set | Pistachio/Bkms_metabolic/Pistachio_ringbreaker/Reaxys/Reaxys_biocatalysis |
Top-N result to add to graph | 6 |
Feasible Synthetic Routes
Q1: How does Bromodichloroacetonitrile compare to other haloacetonitriles in terms of its toxicity to mammalian cells?
A1: [] this compound (BDCAN) exhibits moderate cytotoxicity compared to other haloacetonitriles. The descending rank order for cytotoxicity within this class of compounds, as determined in a study using mammalian cells, is: Tribromoacetonitrile ≈ Dibromoacetonitrile > Bromoacetonitrile ≈ Iodoacetonitrile > Bromochloroacetonitrile ≈ Chlorodibromoacetonitrile > This compound > Dichloroacetonitrile ≈ Chloroacetonitrile ≈ Trichloroacetonitrile. This suggests that the presence and combination of halogen atoms within the haloacetonitrile structure influence its cytotoxic potential. [] Comparative quantitative toxicology and QSAR modeling of the haloacetonitriles: Forcing agents of water disinfection by-product toxicity. (https://www.semanticscholar.org/paper/03754d0de9e8a04afe044b70a999a2ebcb97b44d)
Q2: Can the toxicity of this compound and other related haloacetonitriles be predicted using computational models?
A2: [] Yes, Quantitative Structure-Activity Relationship (QSAR) models have been successfully developed for predicting both the cytotoxicity and genotoxicity of haloacetonitriles, including this compound. These models utilize the compounds' physicochemical parameters and toxicity metrics, offering a valuable tool for assessing the potential hazards of novel haloacetonitriles before conducting in-depth biological evaluations. [] Comparative quantitative toxicology and QSAR modeling of the haloacetonitriles: Forcing agents of water disinfection by-product toxicity. (https://www.semanticscholar.org/paper/03754d0de9e8a04afe044b70a999a2ebcb97b44d)
Disclaimer and Information on In-Vitro Research Products
Please be aware that all articles and product information presented on BenchChem are intended solely for informational purposes. The products available for purchase on BenchChem are specifically designed for in-vitro studies, which are conducted outside of living organisms. In-vitro studies, derived from the Latin term "in glass," involve experiments performed in controlled laboratory settings using cells or tissues. It is important to note that these products are not categorized as medicines or drugs, and they have not received approval from the FDA for the prevention, treatment, or cure of any medical condition, ailment, or disease. We must emphasize that any form of bodily introduction of these products into humans or animals is strictly prohibited by law. It is essential to adhere to these guidelines to ensure compliance with legal and ethical standards in research and experimentation.