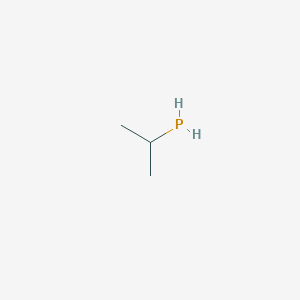
Isopropyl phosphine
Overview
Description
Isopropyl phosphine, specifically isopropyl(phenyl)phosphine (C₉H₁₃P), is an organophosphorus compound featuring a phosphorus atom bonded to an isopropyl group and a phenyl group. Its molecular weight is 152.17 g/mol, and it is utilized in coordination chemistry and catalysis due to its moderate steric bulk and tunable electronic properties . The isopropyl group imparts distinct steric and electronic characteristics, influencing its reactivity and applications in metal-ligand complexes.
Preparation Methods
Synthetic Routes and Reaction Conditions
Isopropyl phosphine can be synthesized through several methods. One common approach involves the reaction of isopropyl halides with phosphine gas. For example, the reaction of isopropyl chloride with phosphine gas in the presence of a base such as sodium or potassium hydroxide can yield this compound . Another method involves the reaction of isopropyl magnesium bromide with phosphorus trichloride, followed by hydrolysis .
Industrial Production Methods
In industrial settings, this compound is typically produced through the reaction of isopropyl alcohol with phosphorus trichloride, followed by reduction with a suitable reducing agent such as lithium aluminum hydride . This method allows for large-scale production of the compound with high purity.
Chemical Reactions Analysis
Types of Reactions
Isopropyl phosphine undergoes various chemical reactions, including:
Reduction: It can be reduced to form this compound hydride using reducing agents like lithium aluminum hydride.
Substitution: This compound can participate in nucleophilic substitution reactions, where it replaces a leaving group in a substrate molecule.
Common Reagents and Conditions
Oxidation: Hydrogen peroxide, oxygen; typically carried out at room temperature.
Reduction: Lithium aluminum hydride; typically carried out under anhydrous conditions.
Substitution: Various alkyl halides; typically carried out in the presence of a base such as sodium hydroxide.
Major Products Formed
Oxidation: this compound oxide.
Reduction: this compound hydride.
Substitution: Various substituted phosphines depending on the substrate used.
Scientific Research Applications
Isopropyl phosphine is a versatile compound with significant applications across chemistry, biology, medicine, and industry, largely due to its strong nucleophilicity, reducing properties, and unique balance of steric properties. It acts as a ligand in organometallic chemistry, facilitating the creation of metal-phosphine complexes, and is used in synthesizing biologically active compounds, such as pharmaceuticals and agrochemicals. Derivatives of this compound are also explored for potential therapeutic uses, including anticancer and antiviral activities.
Scientific Research Applications
- Chemistry: this compound is utilized as a ligand in organometallic chemistry to help form metal-phosphine complexes.
- Biology: It plays a role in synthesizing biologically active compounds, including both pharmaceuticals and agrochemicals.
- Medicine: Derivatives of this compound are being investigated for their potential therapeutic properties, such as anticancer and antiviral activities. Aziridine phosphine oxide derivatives, in particular, have shown noteworthy cell viability inhibition of human cervical epithelioid carcinoma HeLa cells and endometrial adenocarcinoma Ishikawa cells . These compounds disrupt the cell membrane, induce reactive oxygen species (ROS), and cause cell cycle arrest in the S phase .
- Industry: this compound is used in the production of flame retardants and plasticizers, as well as other industrial chemicals.
Case Studies
- Asymmetric Catalysis: P-chirogenic phosphine ligands, synthesized using phosphine-boranes, have been applied in catalytic asymmetric reactions, including mechanistic studies of Rh-catalyzed asymmetric hydrogenation . For example, BisP* and MiniPHOS exhibited high selectivity in catalytic asymmetric syntheses, with selectivity significantly affected by the substituents at the phosphorus atom .
- Nickel(II) Complexes: Tris(1-ethyl-4-iPr-imidazolyl)phosphine (TlEt4iPrIP) was employed for the synthesis of five new nickel(II) complexes: Ni(T1Et4iPrIP)(NCCH3)2(OTf) (1 ), [Ni(T1Et4iPrIP)(OTf)2] (2 ), Ni(T1Et4iPrIP)(H2O)(OTf) (3 ), Ni(T1Et4iPrIP)(Cl) (4 ), and [Ni(T1Et4iPrIP)(Cl)2] (5 ) . These complexes serve as bioinorganic structural model complexes for histidine-coordinated nickel proteins .
- Organocatalysis: Phosphines have been used in organocatalysis, where the initial nucleophilic addition of a phosphine to an electrophilic starting material is a key step . For instance, a multifunctional chiral aminophosphine (P7)-catalyzed enantioselective intramolecular Rauhut–Curtius reaction of cyclohexadienones yielded various hydro-2H-indole derivatives with high yields and excellent stereoselectivities .
- Anticancer Activity: Aziridine phosphine oxides have demonstrated anticancer activity by inhibiting cancer cell viability . Compounds 5 and 7 significantly decreased the cell viability of HeLa and Ishikawa cancer cell lines, with IC50 values comparable to cisplatin . These compounds cause cell arrest in the S phase and increase the number of cells in the sub-G1 phase, indicative of apoptosis .
- Topical Applications: Di-isopropyl-phosphinoyl-alkanes have applications in treating skin discomfort, irritation, itch, and pain .
Data Table
Mechanism of Action
The mechanism of action of isopropyl phosphine involves its strong nucleophilicity and reducing properties. It can donate electrons to electrophilic centers, facilitating various chemical transformations. In biological systems, this compound derivatives can interact with molecular targets such as enzymes and receptors, modulating their activity and leading to therapeutic effects .
Comparison with Similar Compounds
Structural and Steric Properties
Isopropyl phosphine is structurally distinct from other phosphines due to the branched isopropyl substituent. Comparisons with related compounds reveal:
In copper complexes, PiPr₃ exhibits a P–Cu–P angle of ~132°, significantly distorting tetrahedral geometry, whereas PPh₃ maintains near-ideal angles (~109°) .
Electronic and Catalytic Performance
Phosphines with isopropyl groups demonstrate unique electronic properties in catalysis:
- Copolymerization Catalysis : Replacing tert-butyl with isopropyl in phosphine oxide ligands (e.g., catalyst d vs. e ) reduces energy barriers for copolymerization (16.2 vs. higher values), enhancing efficiency in ethylene/vinyl ether copolymer formation .
- Regioselective Hydrosilylation: Isopropyl-containing monodentate phosphines favor anti-Markovnikov products, while bidentate phosphines promote Markovnikov selectivity. The steric profile of isopropyl groups mitigates β-fluorine elimination in trifluoromethyl-containing systems .
- Oxidative Stability : N-Heterocyclic carbenes (NHCs) surpass phosphines in oxidative stability, but isopropyl phosphines remain viable in low-oxidation-state metal complexes (e.g., cobalt-NHC-phosphine hybrids) .
Host-Guest and Solubility Properties
Bulkier phosphines exhibit stronger host-guest interactions:
- Adamantyl-substituted phosphines (AdTPP) have association constants (Kf) twice that of isopropyl or tert-butyl analogs in supercritical CO₂ due to enhanced hydrophobicity .
- Polyimide-Phosphine Hybrids : Isopropylidene-linked polyimides show superior solubility and dielectric properties compared to hexafluoroisopropylidene variants, attributed to the balanced polarity of isopropyl groups .
Thermal and Flame-Retardant Behavior
Phosphine oxide-containing polyimides with isopropyl linkages exhibit:
- Higher thermal stability (decomposition >500°C) due to rigid aromatic backbones.
- Improved flame retardancy (limiting oxygen index >30%) compared to ether-linked analogs, as phosphorus moieties inhibit combustion .
Q & A
Basic Research Questions
Q. What are the established synthetic routes for isopropyl phosphine derivatives, and how do steric hindrance effects influence their preparation?
this compound derivatives (e.g., tri(isopropyl)phosphine) are typically synthesized via sequential Grignard reactions. A two-step approach is required for bulky substituents: bulky Grignard reagents (e.g., i-propyl or cyclohexyl) are added first to minimize steric clashes, followed by smaller reagents (e.g., methyl) . For example, i-propyl(dimethyl)phosphine is synthesized in 55% yield using this method. Key challenges include optimizing reaction temperatures and reagent stoichiometry to avoid side reactions caused by steric bulk.
Q. What spectroscopic and crystallographic techniques are essential for characterizing this compound complexes?
- X-ray crystallography : Critical for determining coordination geometry and bond lengths. For instance, tri(isopropyl)phosphine-AuCN complexes exhibit linear coordination (C–Au–P angle = 177°) with Au–P distances of 2.284 Å .
- NMR spectroscopy : <sup>31</sup>P NMR distinguishes phosphine oxidation states and ligand environments. Chemical shifts correlate with electron-donating/withdrawing effects of substituents.
- Cyclic voltammetry : Used to assess redox behavior. For Mo(VI) complexes with this compound ligands, oxidation potentials shift due to electron-donating i-propyl groups (e.g., -0.12 V vs. SCE) .
Advanced Research Questions
Q. How do steric and electronic properties of this compound ligands modulate catalytic activity in transition metal complexes?
Steric effects are quantified using cone angles (θ), while electronic effects are analyzed via Tolman electronic parameters (TEP). For example:
- Steric threshold: In Mo complexes, a cone angle >135° (calculated for this compound) disrupts catalytic oxo-transfer reactions due to hindered substrate access .
- Electronic modulation: Isopropyl groups lower TEP values (≈2050 cm⁻¹), enhancing metal-to-ligand charge transfer in photochemical pathways . Methodological recommendation : Combine X-ray crystallography (steric analysis) and IR spectroscopy (TEP determination) for systematic ligand optimization.
Q. How can contradictory data on phosphine-containing complexes (e.g., monomeric vs. ionic structures) be resolved?
Contradictions often arise from solvent polarity or crystallization conditions. For example:
- Tri(isopropyl)phosphine-AuCN forms monomeric structures in the solid state (validated by X-ray data) but may ionize in polar solvents . Resolution strategy :
- Conduct parallel experiments in varying solvents (e.g., CH2Cl2 vs. H2O).
- Use conductivity measurements and ESI-MS to detect ionic species in solution.
Q. What advanced computational methods are suitable for predicting this compound ligand behavior in reaction mechanisms?
- DFT calculations : Model transition states in nucleophilic substitution reactions (e.g., SN2 pathways). Isopropyl groups increase activation barriers by 15–20 kJ/mol due to steric hindrance .
- Molecular dynamics (MD) : Simulate ligand flexibility in catalytic cycles. For example, i-propyl rotation in Pd complexes affects substrate binding entropy .
Q. Data Validation and Reproducibility
Q. How should researchers address irreproducible results in phosphine-mediated radical reactions?
Irreproducibility often stems from trace oxygen or moisture. Example:
- Isopropyl diphenylphosphinite forms phosphine oxide under ambient light but yields acridan derivatives under argon with controlled irradiation . Best practices :
- Use Schlenk-line techniques for oxygen-sensitive reactions.
- Validate reaction conditions via in-situ UV-vis spectroscopy to monitor intermediates.
Q. What statistical approaches are recommended for analyzing steric/electronic trends across phosphine ligand libraries?
- Multivariate regression : Correlate cone angles, TEP values, and catalytic outputs (e.g., turnover frequency).
- Principal component analysis (PCA) : Identify dominant factors (e.g., steric vs. electronic) in ligand performance datasets .
Q. Contradictory Findings in Literature
Q. How to reconcile discrepancies in reported phosphine detection methods (e.g., false signals in spectroscopic studies)?
False positives in phosphine detection (e.g., in Venusian atmosphere studies) highlight the need for orthogonal validation:
Properties
IUPAC Name |
propan-2-ylphosphane | |
---|---|---|
Details | Computed by LexiChem 2.6.6 (PubChem release 2019.06.18) | |
Source | PubChem | |
URL | https://pubchem.ncbi.nlm.nih.gov | |
Description | Data deposited in or computed by PubChem | |
InChI |
InChI=1S/C3H9P/c1-3(2)4/h3H,4H2,1-2H3 | |
Details | Computed by InChI 1.0.5 (PubChem release 2019.06.18) | |
Source | PubChem | |
URL | https://pubchem.ncbi.nlm.nih.gov | |
Description | Data deposited in or computed by PubChem | |
InChI Key |
HHDLJTLPOGOXLR-UHFFFAOYSA-N | |
Details | Computed by InChI 1.0.5 (PubChem release 2019.06.18) | |
Source | PubChem | |
URL | https://pubchem.ncbi.nlm.nih.gov | |
Description | Data deposited in or computed by PubChem | |
Canonical SMILES |
CC(C)P | |
Details | Computed by OEChem 2.1.5 (PubChem release 2019.06.18) | |
Source | PubChem | |
URL | https://pubchem.ncbi.nlm.nih.gov | |
Description | Data deposited in or computed by PubChem | |
Molecular Formula |
C3H9P | |
Details | Computed by PubChem 2.1 (PubChem release 2019.06.18) | |
Source | PubChem | |
URL | https://pubchem.ncbi.nlm.nih.gov | |
Description | Data deposited in or computed by PubChem | |
DSSTOX Substance ID |
DTXSID50337030 | |
Record name | Phosphine, (1-methylethyl)- | |
Source | EPA DSSTox | |
URL | https://comptox.epa.gov/dashboard/DTXSID50337030 | |
Description | DSSTox provides a high quality public chemistry resource for supporting improved predictive toxicology. | |
Molecular Weight |
76.08 g/mol | |
Details | Computed by PubChem 2.1 (PubChem release 2021.05.07) | |
Source | PubChem | |
URL | https://pubchem.ncbi.nlm.nih.gov | |
Description | Data deposited in or computed by PubChem | |
CAS No. |
4538-29-8 | |
Record name | Phosphine, (1-methylethyl)- | |
Source | EPA DSSTox | |
URL | https://comptox.epa.gov/dashboard/DTXSID50337030 | |
Description | DSSTox provides a high quality public chemistry resource for supporting improved predictive toxicology. | |
Disclaimer and Information on In-Vitro Research Products
Please be aware that all articles and product information presented on BenchChem are intended solely for informational purposes. The products available for purchase on BenchChem are specifically designed for in-vitro studies, which are conducted outside of living organisms. In-vitro studies, derived from the Latin term "in glass," involve experiments performed in controlled laboratory settings using cells or tissues. It is important to note that these products are not categorized as medicines or drugs, and they have not received approval from the FDA for the prevention, treatment, or cure of any medical condition, ailment, or disease. We must emphasize that any form of bodily introduction of these products into humans or animals is strictly prohibited by law. It is essential to adhere to these guidelines to ensure compliance with legal and ethical standards in research and experimentation.