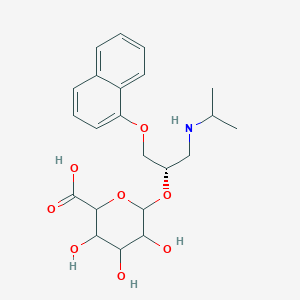
(S)-Propranolol-O-beta-D-glucuronide
- Click on QUICK INQUIRY to receive a quote from our team of experts.
- With the quality product at a COMPETITIVE price, you can focus more on your research.
Overview
Description
(S)-Propranolol-O-beta-D-glucuronide is a metabolite of propranolol, a non-selective beta-adrenergic receptor antagonist widely used in the treatment of cardiovascular diseases. This compound is formed through the glucuronidation of propranolol, a process that enhances its solubility and facilitates its excretion from the body.
Preparation Methods
Synthetic Routes and Reaction Conditions
The synthesis of (S)-Propranolol-O-beta-D-glucuronide typically involves the enzymatic glucuronidation of propranolol. This process is catalyzed by UDP-glucuronosyltransferase enzymes, which transfer glucuronic acid from UDP-glucuronic acid to propranolol. The reaction conditions generally include a buffered aqueous solution at a physiological pH, with the presence of cofactors such as magnesium ions to enhance enzyme activity.
Industrial Production Methods
In an industrial setting, the production of this compound can be scaled up using bioreactors that provide optimal conditions for enzyme activity. These bioreactors maintain a controlled environment, including temperature, pH, and substrate concentration, to maximize the yield of the glucuronide product.
Chemical Reactions Analysis
Types of Reactions
(S)-Propranolol-O-beta-D-glucuronide primarily undergoes hydrolysis, where the glucuronic acid moiety is cleaved off, reverting it back to propranolol. This reaction can occur under acidic or basic conditions.
Common Reagents and Conditions
Hydrolysis: Acidic conditions (e.g., hydrochloric acid) or basic conditions (e.g., sodium hydroxide) are commonly used to hydrolyze this compound.
Oxidation and Reduction: While less common, oxidation and reduction reactions can also occur, depending on the presence of specific oxidizing or reducing agents.
Major Products
The major product of hydrolysis is propranolol, which can further undergo metabolic transformations in the body.
Scientific Research Applications
(S)-Propranolol-O-beta-D-glucuronide has several applications in scientific research:
Pharmacokinetics: Studying the metabolism and excretion of propranolol in the body.
Drug Development: Investigating the role of glucuronidation in drug metabolism and the development of new beta-blockers.
Toxicology: Assessing the safety and potential side effects of propranolol and its metabolites.
Biochemistry: Understanding the enzymatic processes involved in drug metabolism.
Mechanism of Action
(S)-Propranolol-O-beta-D-glucuronide itself does not exert pharmacological effects. Instead, it serves as a metabolite that facilitates the excretion of propranolol. The parent compound, propranolol, works by blocking beta-adrenergic receptors, thereby reducing heart rate, myocardial contractility, and blood pressure. This action is mediated through the inhibition of the sympathetic nervous system.
Comparison with Similar Compounds
Similar Compounds
Propranolol: The parent compound, a non-selective beta-blocker.
Nadolol: Another non-selective beta-blocker with a similar mechanism of action.
Metoprolol: A selective beta-1 adrenergic receptor antagonist.
Uniqueness
(S)-Propranolol-O-beta-D-glucuronide is unique in its role as a metabolite that enhances the solubility and excretion of propranolol. This glucuronidation process is crucial for the detoxification and elimination of many drugs, highlighting the importance of this compound in pharmacokinetics and drug metabolism studies.
Properties
CAS No. |
58657-78-6 |
---|---|
Molecular Formula |
C22H29NO8 |
Molecular Weight |
435.5 g/mol |
IUPAC Name |
(2S,3S,4S,5R,6R)-3,4,5-trihydroxy-6-[(2S)-1-naphthalen-1-yloxy-3-(propan-2-ylamino)propan-2-yl]oxyoxane-2-carboxylic acid |
InChI |
InChI=1S/C22H29NO8/c1-12(2)23-10-14(11-29-16-9-5-7-13-6-3-4-8-15(13)16)30-22-19(26)17(24)18(25)20(31-22)21(27)28/h3-9,12,14,17-20,22-26H,10-11H2,1-2H3,(H,27,28)/t14-,17-,18-,19+,20-,22+/m0/s1 |
InChI Key |
PCALHJGQCKATMK-QBUJOIIVSA-N |
SMILES |
CC(C)NCC(COC1=CC=CC2=CC=CC=C21)OC3C(C(C(C(O3)C(=O)O)O)O)O |
Isomeric SMILES |
CC(C)NC[C@@H](COC1=CC=CC2=CC=CC=C21)O[C@H]3[C@@H]([C@H]([C@@H]([C@H](O3)C(=O)O)O)O)O |
Canonical SMILES |
CC(C)NCC(COC1=CC=CC2=CC=CC=C21)OC3C(C(C(C(O3)C(=O)O)O)O)O |
Origin of Product |
United States |
Retrosynthesis Analysis
AI-Powered Synthesis Planning: Our tool employs the Template_relevance Pistachio, Template_relevance Bkms_metabolic, Template_relevance Pistachio_ringbreaker, Template_relevance Reaxys, Template_relevance Reaxys_biocatalysis model, leveraging a vast database of chemical reactions to predict feasible synthetic routes.
One-Step Synthesis Focus: Specifically designed for one-step synthesis, it provides concise and direct routes for your target compounds, streamlining the synthesis process.
Accurate Predictions: Utilizing the extensive PISTACHIO, BKMS_METABOLIC, PISTACHIO_RINGBREAKER, REAXYS, REAXYS_BIOCATALYSIS database, our tool offers high-accuracy predictions, reflecting the latest in chemical research and data.
Strategy Settings
Precursor scoring | Relevance Heuristic |
---|---|
Min. plausibility | 0.01 |
Model | Template_relevance |
Template Set | Pistachio/Bkms_metabolic/Pistachio_ringbreaker/Reaxys/Reaxys_biocatalysis |
Top-N result to add to graph | 6 |
Feasible Synthetic Routes
Disclaimer and Information on In-Vitro Research Products
Please be aware that all articles and product information presented on BenchChem are intended solely for informational purposes. The products available for purchase on BenchChem are specifically designed for in-vitro studies, which are conducted outside of living organisms. In-vitro studies, derived from the Latin term "in glass," involve experiments performed in controlled laboratory settings using cells or tissues. It is important to note that these products are not categorized as medicines or drugs, and they have not received approval from the FDA for the prevention, treatment, or cure of any medical condition, ailment, or disease. We must emphasize that any form of bodily introduction of these products into humans or animals is strictly prohibited by law. It is essential to adhere to these guidelines to ensure compliance with legal and ethical standards in research and experimentation.