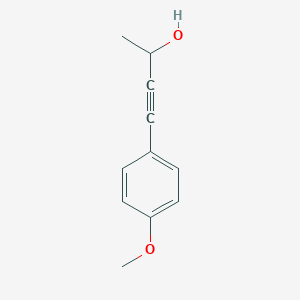
4-(4-Methoxyphenyl)but-3-yn-2-ol
- Click on QUICK INQUIRY to receive a quote from our team of experts.
- With the quality product at a COMPETITIVE price, you can focus more on your research.
Overview
Description
4-(4-Methoxyphenyl)but-3-yn-2-ol is an organic compound with the molecular formula C₁₁H₁₂O₂ It is characterized by the presence of a methoxyphenyl group attached to a butyn-2-ol backbone
Preparation Methods
Synthetic Routes and Reaction Conditions: The synthesis of 4-(4-Methoxyphenyl)but-3-yn-2-ol typically involves the reaction of 4-iodoanisole with 3-butyn-2-ol under specific conditions. The reaction is often catalyzed by palladium and copper catalysts in the presence of a base such as potassium carbonate. The reaction is carried out in an inert atmosphere, usually under nitrogen or argon, to prevent oxidation .
Industrial Production Methods: Industrial production methods for this compound are similar to laboratory synthesis but are scaled up to accommodate larger quantities. The use of continuous flow reactors and optimized reaction conditions can enhance yield and efficiency. Solvent-free conditions and green chemistry principles are often employed to minimize environmental impact .
Chemical Reactions Analysis
Types of Reactions: 4-(4-Methoxyphenyl)but-3-yn-2-ol undergoes various chemical reactions, including:
Oxidation: The compound can be oxidized to form corresponding ketones or aldehydes.
Reduction: Reduction reactions can convert the alkyne group to an alkene or alkane.
Substitution: The methoxy group can be substituted with other functional groups under appropriate conditions.
Common Reagents and Conditions:
Oxidation: Common oxidizing agents include potassium permanganate (KMnO₄) and chromium trioxide (CrO₃).
Reduction: Catalytic hydrogenation using palladium on carbon (Pd/C) or lithium aluminum hydride (LiAlH₄) can be employed.
Substitution: Nucleophilic substitution reactions often use reagents like sodium hydride (NaH) or organolithium compounds.
Major Products Formed:
Oxidation: Formation of 4-(4-methoxyphenyl)but-3-yn-2-one.
Reduction: Formation of 4-(4-methoxyphenyl)but-3-ene-2-ol or 4-(4-methoxyphenyl)butane-2-ol.
Substitution: Formation of various substituted derivatives depending on the nucleophile used.
Scientific Research Applications
4-(4-Methoxyphenyl)but-3-yn-2-ol has diverse applications in scientific research:
Mechanism of Action
The mechanism of action of 4-(4-Methoxyphenyl)but-3-yn-2-ol involves its interaction with specific molecular targets and pathways. The compound can act as an inhibitor or activator of certain enzymes, depending on its structure and functional groups. The methoxy group and the alkyne moiety play crucial roles in its reactivity and interaction with biological molecules .
Comparison with Similar Compounds
4-Ethynylanisole: Similar structure but lacks the hydroxyl group.
4-Methoxybenzaldehyde: Contains a methoxy group but has an aldehyde instead of an alkyne.
3-Butyn-2-ol: Similar backbone but lacks the methoxyphenyl group.
Uniqueness: 4-(4-Methoxyphenyl)but-3-yn-2-ol is unique due to the combination of a methoxyphenyl group and an alkyne moiety, which imparts distinct chemical and biological properties.
Properties
CAS No. |
111887-18-4 |
---|---|
Molecular Formula |
C11H12O2 |
Molecular Weight |
176.21 g/mol |
IUPAC Name |
4-(4-methoxyphenyl)but-3-yn-2-ol |
InChI |
InChI=1S/C11H12O2/c1-9(12)3-4-10-5-7-11(13-2)8-6-10/h5-9,12H,1-2H3 |
InChI Key |
SEPVVYYGLVIZMG-UHFFFAOYSA-N |
Canonical SMILES |
CC(C#CC1=CC=C(C=C1)OC)O |
Origin of Product |
United States |
Disclaimer and Information on In-Vitro Research Products
Please be aware that all articles and product information presented on BenchChem are intended solely for informational purposes. The products available for purchase on BenchChem are specifically designed for in-vitro studies, which are conducted outside of living organisms. In-vitro studies, derived from the Latin term "in glass," involve experiments performed in controlled laboratory settings using cells or tissues. It is important to note that these products are not categorized as medicines or drugs, and they have not received approval from the FDA for the prevention, treatment, or cure of any medical condition, ailment, or disease. We must emphasize that any form of bodily introduction of these products into humans or animals is strictly prohibited by law. It is essential to adhere to these guidelines to ensure compliance with legal and ethical standards in research and experimentation.