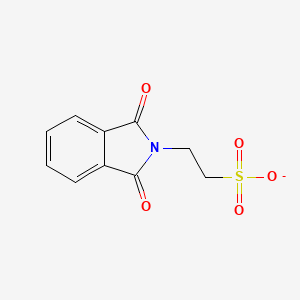
1,3-Dioxo-2-isoindolineethanesulfonate
- Click on QUICK INQUIRY to receive a quote from our team of experts.
- With the quality product at a COMPETITIVE price, you can focus more on your research.
Overview
Description
2-(1,3-dioxo-1,3-dihydro-2H-isoindol-2-yl)ethanesulfonate potassium is a chemical compound that belongs to the class of isoindoline derivatives. This compound is characterized by the presence of a sulfonate group attached to an isoindoline ring, which is further substituted with a dioxo group. It is often used in various scientific research applications due to its unique chemical properties.
Preparation Methods
Synthetic Routes and Reaction Conditions
The synthesis of 2-(1,3-dioxo-1,3-dihydro-2H-isoindol-2-yl)ethanesulfonate potassium typically involves the reaction of phthalimide with ethanesulfonyl chloride in the presence of a base such as potassium carbonate. The reaction is carried out in an organic solvent like dichloromethane at room temperature. The product is then purified through recrystallization or chromatography to obtain the desired compound in high purity.
Industrial Production Methods
On an industrial scale, the production of this compound follows similar synthetic routes but with optimized reaction conditions to enhance yield and reduce production costs. The use of continuous flow reactors and automated purification systems are common in industrial settings to ensure consistent quality and scalability.
Chemical Reactions Analysis
Types of Reactions
2-(1,3-dioxo-1,3-dihydro-2H-isoindol-2-yl)ethanesulfonate potassium undergoes various chemical reactions, including:
Oxidation: The compound can be oxidized to form sulfonic acid derivatives.
Reduction: Reduction reactions can convert the dioxo group to hydroxyl groups.
Substitution: The sulfonate group can be substituted with other nucleophiles under appropriate conditions.
Common Reagents and Conditions
Oxidation: Common oxidizing agents include potassium permanganate and hydrogen peroxide.
Reduction: Reducing agents such as sodium borohydride and lithium aluminum hydride are used.
Substitution: Nucleophiles like amines and thiols can be used in substitution reactions, often in the presence of a catalyst.
Major Products Formed
Oxidation: Sulfonic acid derivatives.
Reduction: Hydroxyl-substituted isoindoline derivatives.
Substitution: Various substituted isoindoline derivatives depending on the nucleophile used.
Scientific Research Applications
2-(1,3-dioxo-1,3-dihydro-2H-isoindol-2-yl)ethanesulfonate potassium is utilized in several scientific research fields:
Chemistry: Used as a reagent in organic synthesis and as a building block for more complex molecules.
Biology: Employed in the study of enzyme inhibition and protein interactions.
Medicine: Investigated for its potential therapeutic properties, including anti-inflammatory and anticancer activities.
Industry: Used in the production of specialty chemicals and materials.
Mechanism of Action
The mechanism of action of 2-(1,3-dioxo-1,3-dihydro-2H-isoindol-2-yl)ethanesulfonate potassium involves its interaction with specific molecular targets, such as enzymes and receptors. The compound can inhibit enzyme activity by binding to the active site or allosteric sites, thereby altering the enzyme’s conformation and function. Additionally, it can interact with cellular receptors, modulating signal transduction pathways and affecting cellular responses.
Comparison with Similar Compounds
Similar Compounds
- 2-(1,3-dioxo-1,3-dihydro-2H-isoindol-2-yl)propanoic acid
- N-Phthalyl-DL-alanine
- 2-phthalimidopropionic acid
Uniqueness
Compared to similar compounds, 2-(1,3-dioxo-1,3-dihydro-2H-isoindol-2-yl)ethanesulfonate potassium is unique due to the presence of the sulfonate group, which imparts distinct solubility and reactivity properties. This makes it particularly useful in applications where water solubility and specific reactivity are required.
Properties
IUPAC Name |
2-(1,3-dioxoisoindol-2-yl)ethanesulfonate |
Source
|
---|---|---|
Details | Computed by Lexichem TK 2.7.0 (PubChem release 2021.05.07) | |
Source | PubChem | |
URL | https://pubchem.ncbi.nlm.nih.gov | |
Description | Data deposited in or computed by PubChem | |
InChI |
InChI=1S/C10H9NO5S/c12-9-7-3-1-2-4-8(7)10(13)11(9)5-6-17(14,15)16/h1-4H,5-6H2,(H,14,15,16)/p-1 |
Source
|
Details | Computed by InChI 1.0.6 (PubChem release 2021.05.07) | |
Source | PubChem | |
URL | https://pubchem.ncbi.nlm.nih.gov | |
Description | Data deposited in or computed by PubChem | |
InChI Key |
GSLVEXPADWBUAC-UHFFFAOYSA-M |
Source
|
Details | Computed by InChI 1.0.6 (PubChem release 2021.05.07) | |
Source | PubChem | |
URL | https://pubchem.ncbi.nlm.nih.gov | |
Description | Data deposited in or computed by PubChem | |
Canonical SMILES |
C1=CC=C2C(=C1)C(=O)N(C2=O)CCS(=O)(=O)[O-] |
Source
|
Details | Computed by OEChem 2.3.0 (PubChem release 2021.05.07) | |
Source | PubChem | |
URL | https://pubchem.ncbi.nlm.nih.gov | |
Description | Data deposited in or computed by PubChem | |
Molecular Formula |
C10H8NO5S- |
Source
|
Details | Computed by PubChem 2.1 (PubChem release 2021.05.07) | |
Source | PubChem | |
URL | https://pubchem.ncbi.nlm.nih.gov | |
Description | Data deposited in or computed by PubChem | |
Molecular Weight |
254.24 g/mol |
Source
|
Details | Computed by PubChem 2.1 (PubChem release 2021.05.07) | |
Source | PubChem | |
URL | https://pubchem.ncbi.nlm.nih.gov | |
Description | Data deposited in or computed by PubChem | |
Disclaimer and Information on In-Vitro Research Products
Please be aware that all articles and product information presented on BenchChem are intended solely for informational purposes. The products available for purchase on BenchChem are specifically designed for in-vitro studies, which are conducted outside of living organisms. In-vitro studies, derived from the Latin term "in glass," involve experiments performed in controlled laboratory settings using cells or tissues. It is important to note that these products are not categorized as medicines or drugs, and they have not received approval from the FDA for the prevention, treatment, or cure of any medical condition, ailment, or disease. We must emphasize that any form of bodily introduction of these products into humans or animals is strictly prohibited by law. It is essential to adhere to these guidelines to ensure compliance with legal and ethical standards in research and experimentation.